- Home
- Assessments
- Bioregional Assessment Program
- Namoi subregion
- 2.1-2.2 Data analysis for the Namoi subregion
- 2.1.5 Surface water – groundwater interactions
- 2.1.5.4 Statistical analysis and interpolation
A new surface water – groundwater connectivity assessment representative of 2012 baseline conditions in the Namoi subregion was undertaken to help inform the surface water and groundwater numerical modelling components of the BA. The datasets and data processing methods that were used to develop the 2012 baseline assessment of surface water – groundwater connectivity and key findings are presented in the following sections.
2.1.5.4.1 Methods
A basin-scale surface water – groundwater connectivity assessment was undertaken for June 2012, and included the following:
- development of potentiometric surface of watertable aquifers
- determination of depth to watertable
- assessment of surface water – groundwater connectivity based on groundwater levels.
The basin-scale connectivity analysis was followed by a hydrological analysis of the Mooki river basin to provide additional process understanding of surface water – groundwater connectivity in this area. The hydrological analysis utilised streamflow data over the 2003 to 2012 period. Flow characteristics (stream hydrograph, baseflow component and flow duration data) were used to infer the dominant direction of flux over the assessment period and the findings were compared with pre-2003 conditions. Differential gauging results in the Mooki river basin for 2006, representing a dry period, and 2012, representing a wet period, were assessed and compared. Finally, in order to better conceptualise the changes in the watertable over time and provide greater context to the 2012 baseline surface water – groundwater connectivity assessment, the changes in the depth to watertable between 2006 and 2012 were evaluated. Further details and discussion follow in the sections below.
2.1.5.4.2 Namoi region basin-scale connectivity assessment
Raster surfaces representing the potentiometric surface and depth to groundwater were created using ArcGIS based on data obtained from monitoring bores screening the shallow alluvial aquifers (less than or equal to 45 m deep) using data for June 2012, or as close as possible (Bioregional Assessment Programme, Dataset 1). The month of June was chosen to enable comparison to the CSIRO June 2006 (CSIRO, 2007) investigation, with June typically being a low-flow month outside of irrigation season influences that commonly occur during the September to March period.
The water elevation point data for the shallow alluvial aquifers were used to create an interpolated potentiometric surface (groundwater level) (Figure 34), and shows that groundwater flows from the eastern margins in a north and westerly direction within the Namoi subregion, generally consistent with the surface water flow direction. Groundwater gradients are steeper, with contours located closer together, in the upper catchment where the topography is more elevated and steep and in areas of bedrock highs which constrict groundwater flow.
Figure 35 shows the locations of monitoring bores in the shallow alluvium (less than or equal to 45 m) together with the associated depth to watertable in June 2012, or as close as possible. These point data were used to create an interpolated depth to watertable raster surface using ArcGIS (Figure 36).
Figure 34 Watertable level interpolated surface for the Namoi subregion, June 2012
Data: Bioregional Assessment Programme (Dataset 2)
Data: Bioregional Assessment Programme (Dataset 3)
Figure 36 Depth to watertable interpolated surface for the shallow alluvial aquifers, June 2012
Data: Bioregional Assessment Programme (Dataset 2)
2.1.5.4.3 Inferring surface water – groundwater connectivity from groundwater levels
Monitoring bore data from within 1 km of the streams in the Namoi subregion were used to create a near-stream watertable raster surface representing June 2012 conditions (Figure 36). The data are summarised in Table 10. The same methodology used by Ivkovic (2006) to assess surface water – groundwater connectivity was employed, using a depth of 10 m as the cut-off point at which connectivity was inferred (Figure 38). Using a similar methodology allowed for a direct comparison of the changes in connectivity between the time of the Ivkovic (2006) investigation, representing groundwater level data from a time when there was less agricultural and coal resource development, relative to June 2012.
Recent field studies by Brownbill et al. (2011) in the Murray-Darling Basin, including two small field sites in the Lower Namoi river basin (Old Mollee and Yarral East), have suggested that subsurface depth of surface water – groundwater connectivity may be less than 10 m. The Brownbill et al. (2011) study indicated that a cut-off of 6 m depth to water in the riparian zone may be more appropriate for determining connectivity status at lower elevations, where the topographic variation is relatively subdued. However, there is an absence of catchment-wide data in the riparian zone to confirm this depth more generally. Therefore, the use of 10 m for regional-scale mapping was considered appropriate for the BA for the Namoi subregion.
An additional class representing depths to groundwater in the range of greater than 10 to 12 m has been added to the connectivity map (Figure 38) to highlight stream reaches that are interpreted to transition from connected to disconnected. Although the addition of a transition class is somewhat arbitrary with respect to the depth to watertable range selected, this class was added to emphasise that streams transition from a state of connectivity to disconnection, rather than there being an abrupt change in the state of connection at some point along a stream reach. Moreover, an additional depth of 2 m over the 10 m connectivity cut-off depth allows for the importance of subsurface storages of water in the unsaturated zone in maintaining connectivity, where capillary effects are commonly reported to 2 m thickness (Kuo, 1999). Also, a transitional class is useful for indicating areas where the infiltration rate, or stream loss, to the underlying groundwater system may still be influenced by changes in watertable depth (Brownbill et al., 2011). Importantly, the transition to disconnection class also attempts to account for the sparse monitoring networks along the streams, which may not adequately represent underlying recharge mounds beneath the streams. These recharge mounds are critical to maintaining connectivity in losing stream systems (Lamontagne et al., 2014). These assumptions regarding the relationship between depth to groundwater and connectivity are made in the absence of more detailed peri-stream aquifer–streambed surveys that would allow for more robust assessment of connectivity in the Namoi river basin.
Data: Bioregional Assessment Programme (Dataset 2)
Connected surface water – groundwater reaches (< or = 10 m); transition reaches (>10 m to 12 m); disconnected reaches (>12 m)
Data: Bioregional Assessment Programme (Dataset 2)
Table 10 List of shallow monitoring bores located within 1 km of the stream and their water levels in June 2012, or as close as possible
Data: Bioregional Assessment Programme (Dataset 4)
The surface water – groundwater connectivity assessment in Figure 38, when compared to the assessments previously made by CSIRO (2007) (Figure 30) and Ivkovic (2006) (Figure 32), indicates an increase in regions of disconnection where the interpolated depth to watertable is greater than 10 m. Areas of increased disconnection occur in the Mooki river basin, in the region from Quirindi downstream to Caroona, and a small portion of the river reach between Breeza and Gunnedah. Reaches classed as transitional occur in the mid Mooki river basin downstream of Breeza and small areas in the Coxs creek basin, both upstream and downstream of the disconnected reach between Mullaley and Boggabri. This suggests a greater proportion of stream reach is becoming disconnected with a lowering of the watertable. Another area in the Upper Namoi river basin with disconnected and transition to disconnection reaches is the region around Carroll. The assumptions in this assessment include:
- a depth to watertable of less than or equal to 10 m indicates an area of probable hydraulic connection between the aquifer and stream
- a class of greater than 10 to 12 m indicates a transition to disconnection class
- a depth to watertable of greater than 12 m indicates an area of probable disconnection
- the interpolated surface obtained from monitoring bores located within 1 km of the streams accurately represents the watertable level adjacent to the stream in June 2012, or as close as possible.
2.1.5.4.4 Hydrological analysis of Mooki river basin
The Mooki river basin hosts a number of historical and proposed coal mines including the Caroona and Watermark projects (refer to companion product 1.2 (coal and coal seam gas resource assessment) (Northey et al., 2014) and product 2.3 (conceptual modelling) for the Namoi subregion (Herr et al., 2018)). The more detailed analysis here is undertaken to assess surface water – groundwater interactions in this catchment and to inform hydrological modelling, both surface water and groundwater (refer to companion product 2.6.1 (Aryal et al., 2018) and product 2.6.2 (Janardhanan et al., 2018) for the Namoi subregion).
In order to further assess connectivity in the Mooki river basin, hydrometric data were analysed to cross-validate the interpolated depth to watertable surface and connectivity interpretation. Figure 38 shows the Mooki river basin and the locations of the streamflow gauging stations used in this analysis. The streamflow gauging station data were analysed over the period May 2003 to July 2012 (9.1 years) to assess how surface water – groundwater interactions during this period differed to the pre-2003 (Ivkovic, 2006) investigation. This date range was selected because data were available across all streamflow gauging stations without any gaps. It is noted that the pre-2003 period does not represent an undisturbed system, as declines in groundwater levels were already being observed at this time. For example CSIRO (2007) showed increasingly losing conditions over the period 1997 to 2007.
The observed streamflow was filtered using the Lyne and Hollick (1979) baseflow filter using a filter parameter of 0.925. Flow characteristics were assessed according to the shape of the streamflow hydrograph (an example hydrograph is shown in Figure 40) as well as flow duration data (Figure 41) using the methods described in Section 2.1.5.2.2 to determine the dominant direction of flux over the assessment period, with characteristics summarised in Table 11.
It is noted that baseflow analysis contains a number of assumptions (Halford and Mayer, 2000) and further investigation of the baseflow characteristics of the Namoi river basin would be a useful contribution to future studies.
Figure 39 Streamflow gauging stations analysed in the Mooki river basin
Connected-gaining stream reaches are classified (in this study) as those with flow measured over 90% of the streamflow record, and where analysis of groundwater levels and stream height indicate connection (Figure 38).
Data: Bioregional Assessment Programme (Dataset 5)
Data: Bioregional Assessment Programme (Dataset 6)
When compared to the pre-2003 period, the mean flows (total discharge and baseflow) between 2003 and 2012 are considerably reduced (Table 11). The percentage difference reductions in mean baseflow of the order of 62% and 20% were calculated for gauging stations 419027 and 419084 respectively. The reduction in flows is likely to be in part due to the lower than average rainfall since 2003, as well as the influence of groundwater extractions on river connectivity and flows. Overall, there is a similar pattern as described by Ivkovic (2006) with baseflows generated in the upper catchment, with a decrease in the baseflow index (BFI) and flow duration down the catchment.
Some sections of the Mooki River between gauging stations 419027 and 419084 were mapped as transition to disconnected reaches, based on the interpolated depth to watertable (Figure 38). The streamflow data suggest that this section of the river has maintained variable connection as a mostly losing reach between 2003 and 2012, and this represents a change from being identified as a mostly gaining reach over the length of the hydrological data record prior to 2003.
Table 11 Flow characteristics at unregulated streamflow gauging stations in the Mooki river basin
aBased on visual inspection of the stream hydrograph and flow duration data using Ivkovic (2009) methodology
bNo baseflows are expected at this site because the river is disconnected from the underlying groundwater.
cThe estimated BFI of 0.07 gives an indication of the range of error possible when using the Lyne and Hollick filter using a filter parameter of 0.925 in this subcatchment.
Data: Bioregional Assessment Programme (Dataset 7)
There are few monitoring bores near the streamflow gauging stations along the Mooki River by which to confirm groundwater levels adjacent to the river. Further assessment of surface water – groundwater connectivity between Caroona and Breeza or groundwater management zone 8 more generally is warranted given the water resource pressures in this zone.
The current hydrological assessment confirms the connectivity mapping in the river around gauging station 419098, as a disconnected section along Quirindi Creek, between Caroona and Quirindi (Figure 38), as suggested by the absence of baseflow periods in the stream hydrograph, and the steep slope of the flow duration data (Figure 41). This area had been previously mapped as a connected aquifer–river system by Ivkovic (2006).
Differential gauging results for 2012 and 2006 in the Mooki river basin
The differences in mean river discharge between gauging stations 419034, 419027 and 419084 were calculated for the 2012 (wet year) and 2006 (dry year) assessment periods to estimate the mean volume of water lost or gained along the river reach (between the streamflow gauging stations). It was assumed for this estimation that evapotranspiration effects and inflows from the ephemeral Quirindi Creek and other minor tributaries were negligible. The flow summary statistics presented in Table 11 and Table 12 suggest that while this is a simplification of the system, it is not unreasonable. Quirindi Creek flowed only 13% of the time in the period 2003 to 2012, and mean discharge over this period was less than 20% of the mean discharge in the Mooki River upstream of the confluence of the two streams.
The results for the 2012 wet year show transmission losses of around 1.2 ML/day/km between gauging stations 419034 and 419027, followed by a subsequent gain of approximately 20 ML/day/km between gauging stations 419027 and 419084 (Table 12). Overall, there was a cumulative gain of 12.9 ML/day/km between the upstream gauge (419034) through to the catchment outlet as measured at Ruvigne (419084).
Table 12 Differential gauging results for mean flows in the Mooki river basin for 2006 (dry year) and 2012 (wet year)
aminus = gain, na = not applicable
Data: Bioregional Assessment Programme (Dataset 8)
The results for the 2006 dry year show transmission losses along the length of river from gauging station 419034 through to 419084 (Table 12). The mean losses estimated at gauging station 419084 are very low due to the extremely dry conditions in 2006, resulting in the river being dry throughout much of the year.
The differential gauging results demonstrate how the Mooki River reaches vary in their dominant flux, with river losses dominating over dry periods and gains over wet periods. River losses between gauging stations 419034 and 419027 now occur during wet periods as well as dry, with data from gauge 419027 indicating a change from a variably connected, mostly gaining system to a mostly losing system as an aggregate upstream response. The data from gauging station 419084 suggest a variably connected mostly losing river reach as an aggregate upstream response, consistent with pre-2003 conditions.
This analysis could be further refined using data for the metered extraction bores and river off-takes in the area of analysis. This would enable a more detailed analysis of the losses between gauges, to take account of extractions. However, the required data were not available for this bioregional assessment.
2.1.5.4.5 Groundwater level changes between 2006 and 2012
To better understand the changes in depth to watertable over time, and to provide context to the 2012 baseline connectivity investigation, groundwater level (depth to watertable) changes were assessed between 2006 and 2012. The year 2006 was used as a comparison year with 2012 for several reasons, including:
- The CSIRO (2007) investigation represents a snapshot in time for the June 2006 period.
- The year 2006 was when the Water Sharing Plans for the Upper and Lower Namoi Groundwater Sources were initially implemented (NSW Department of Primary Industries Office of Water, 2013), so the influence of these plans between 2006 and 2012 can be assessed, to some extent.
- The 2006 period represents a period of drought, which lasted through to 2009. This dry period was subsequently followed by drought breaking conditions between 2010 through to 2012, when annual rainfall totals were significantly and consistently higher than average across the entire Namoi river basin (Burrell et al., 2013).
The residual mass rainfall curve for data from the Boggabri Post Office (gauging station 55007) between 1970 and 2012 is shown in Figure 42. The residual mass represents the cumulative deviation from the mean monthly rainfall (blue line) over the length of the rainfall record assessed (orange line), with the falling sections of the residual mass (green line) indicating periods of below average rainfall.
Data: Bureau of Meteorology (Dataset 9)
A comparison of the 2006 and 2012 conditions allows for an assessment to be made on how groundwater storages have changed during the 2010 to 2012 high rainfall years from the previous drought years. The difference between the 2012 and 2006 depth to watertable interpolated raster surfaces for the shallow aquifer is shown in Figure 43. The figure also shows the locations of monitoring bores used to create the interpolated surfaces, highlighting areas where input data are limited (for example upstream of Caroona), or single data points have a strong influence on the predicted surface (for example in the lower Namoi). Figure 43 shows widespread increases in watertable level over large areas of the Namoi river basin, likely indicating replenishment of groundwater resources during higher rainfall years. Large increases in the watertable level, by up to 11 m in some areas, are shown in areas of the Mooki river basin (between Breeza and Gunnedah) and the Coxs creek basin (just to the north of Mullaley), where the streams have been mapped (in the 2012 assessment) as transition to disconnection and disconnected reaches (Figure 38). Despite the evidence of considerable increases in watertable level between 2006 and 2012, the increases in groundwater storage have not been sufficiently high to return transitional and disconnected stream reaches to the previous state of connection (based on earlier interpretations).
The area between Caroona and Breeza in the Mooki river basin (groundwater management zone 8, refer to Figure 33) shows only modest levels of groundwater recovery (0.8 m or less) between 2006 and 2012, as evidenced by a typical bore hydrograph GW030008 (located about 3 km south-east of the Mooki River) (Figure 44). One can see from this figure that the water levels exhibit a pattern indicative of regular perturbation that appears to reflect groundwater extraction events, as well as a response to rainfall trends. It is evident from this hydrograph that groundwater levels were at their highest in the 1970s, and that there has been a decline in water levels by more than 10 m within the shallow aquifer (Pipe 1) since then. These declines in the regional watertable level are likely to have impacted on surface water – groundwater connectivity in groundwater management zone 8.
Further analysis of Figure 44 suggests that extractions from the deeper (Gunnedah Formation) aquifer appear to be inducing downward flows from the upper (Narrabri Formation) aquifer. Barrett (2012) reported that extraction from the Gunnedah Formation was inducing downward leakage from the overlying Narrabri Formation in many parts of the Upper Namoi river basin, and that in some areas, this was resulting in dewatering of the upper aquifer. Ivkovic (2006) also reported on the apparent widespread incidence of induced downward leakage as a consequence of groundwater extraction from deeper aquifers based on the analysis of nested bore hydrographs.
The pressures in the deepest aquifer represented by Pipe 3 (50.6–56.7 m screened interval; Figure 44), are typically more elevated than those in Pipe 1 and 2 during recharge/water level recovery events, indicating an upward vertical pressure gradient. This agrees with the research of Lavitt (1999), who analysed water samples from the Mooki river basin and found that the samples from the deeper alluvial units were more similar in composition to the surrounding fractured rock aquifer than the upper alluvium. Based on the hydrochemical data, Lavitt (1999) reported that the deeper alluvium and shallow fractured rock aquifers appear to be hydraulically connected.
Widespread areas of groundwater level decline, shown in orange and red in Figure 43, occur in the Lower Namoi alluvial groundwater source downstream of Narrabri. These declines may be a result of a range of factors, including time lag effects associated with groundwater extractions from the Upper Namoi aquifers over previous decades, which have resulted in reduced throughflow volumes into the Lower Namoi aquifers, in combination with the current levels of extraction in the Lower Namoi. These factors warrant further investigation.
Data: Bureau of Meteorology (Dataset 2)
This figure shows water level data for a nested piezometer monitoring bore site. Pipe 1 screens the Narrabri Formation, Pipe 2 screens the Gunnedah Formation and Pipe 3 screens a mix of lower Gunnedah Formation and fractured rock basalt. (Water levels shown are relative to the measuring point.)
Data: Bureau of Meteorology (Dataset 10)
2.1.5.4.6 Fractured rock aquifer connectivity
Although small-scale groundwater extraction, on the order of approximately 1,000 ML/month between January 2002 and December 2009 (SWS, 2012), occurs from the underlying consolidated rocks surrounding the alluvium in the Namoi river basin (compared to 17,200 ML/month from the alluvium), these rock layers generally make poor aquifers because of their relatively low yields and generally poor quality water. Therefore, there are limited data available by which to characterise them.
Ivkovic (2006) demonstrated widespread connectivity between the shallow and deeper alluvial aquifers, as well as an upward pressure gradient from the underlying fractured rocks in some areas of the Coxs creek and Mooki river basins (see Figure 44 for example). However, the extent of vertical connectivity is unknown.
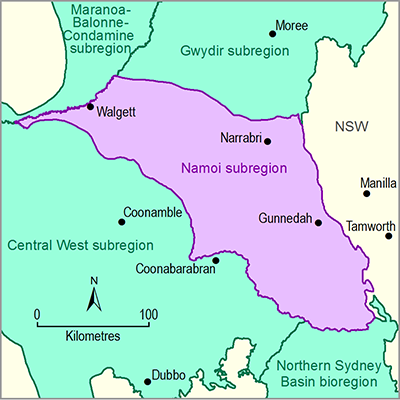
Product Finalisation date
- 2.1.1 Geography
- 2.1.2 Geology
- 2.1.3 Hydrogeology and groundwater quality
- 2.1.4 Surface water hydrology and water quality
- 2.1.5 Surface water – groundwater interactions
- 2.1.5.1 Observed data
- 2.1.5.2 Previous catchment-scale investigations on stream-aquifer interactions
- 2.1.5.3 Overview of controls on surface water – groundwater connectivity based on previous investigations in the Namoi river basin
- 2.1.5.4 Statistical analysis and interpolation
- 2.1.5.5 Gaps
- References
- Datasets
- 2.1.6 Water management for coal resource developments
- 2.1.6.1 Boggabri Coal Mine (baseline) and Boggabri Coal Expansion Project (ACRD)
- 2.1.6.2 Narrabri North Mine (baseline)
- 2.1.6.3 Narrabri South Project (ACRD)
- 2.1.6.4 Rocglen Mine (baseline)
- 2.1.6.5 Sunnyside Mine (baseline)
- 2.1.6.6 Tarrawonga Mine (baseline) and Tarrawonga Coal Expansion Project (ACRD)
- 2.1.6.7 Caroona Coal Project (ACRD)
- 2.1.6.8 Maules Creek Project (ACRD)
- 2.1.6.9 Watermark Coal Project (ACRD)
- 2.1.6.10 Vickery Coal Project (ACRD)
- 2.1.6.11 Narrabri Gas Project (ACRD)
- 2.1.6.12 Mine footprints
- References
- Datasets
- Citation
- Acknowledgements
- Currency of scientific results
- Contributors to the Technical Programme
- About this technical product