Numerous researchers have investigated the origin and composition of coal seam gas (CSG) in eastern Australian basins. According to Smith et al. (1992, p. 63), Smith (1999) and Ahmed and Smith (2001, p. 814), wet thermogenic gases, which initially charged the Sydney Basin were lost due to uplift and erosion, and were almost completely replaced by very dry biogenic methane (CH4) and magmatic carbon dioxide (CO2). The origin of most of the methane in the Sydney Basin can be attributed to the microbial reduction of primarily magmatic carbon dioxide. Evidence for large scale igneous injections of carbon dioxide into coal measure sequences of the Sydney Basin has been provided by Baker et al. (1995), and further supported by Golab et al. (2006, p. 296) in a study on dawsonite formation in coals of the Hunter Coalfield.
Burra et al. (2014, p. 448) provided an alternative Sydney Basin gas model based on research into groundwater chemistry and the behaviour of dissolved gases such as carbon dioxide. According to their observations the central and eastern parts of the basin do not host spatially extensive carbon dioxide gas accumulations whereas northern, western and southern parts of the basin commonly contain CO2‑rich gases at depth. These researchers suggested that this was derived from local- to continental-scale magmatic intrusions and is a product of carbonate dissolution or acetate fermentation.
Craig (2005) investigated CSG distribution in the Hunter Coalfield using gas data from six boreholes drilled between 1991 and 2004. Three of the boreholes were drilled west of the Mount Ogilvie Fault, one to the east of the Bayswater Syncline, and two in the south-west of the coalfield. It was reported that elevated gas contents of around 24.6 m3/t are present in the south, where gas contents vary from about 1.0 m3/t in the upper seams of the Jerrys Plains Subgroup to greater than 24.0 m3/t in the Vane Subgroup (Craig, 2005). Gas contents in the Jerrys Plains Subgroup and upper seams of the Vane Subgroup are noticeably lower in the north than in the south of the coalfield, ranging from approximately 8.0 m3/t at 200 m depth, to a maximum of 14.8 m3/t at about 300 m depth. Coals in the Muswellbrook region show even lower gas contents (Craig, 2005). The study reported that carbon dioxide content increases with depth in the south of the coalfield from 11% at 350 m depth to 84% at 700 m depth. Similarly in the north, carbon dioxide increases with increasing depth, although not consistently for the entire area. Other trends in gas distribution reported by Craig (2005) include observations that no significant relationship could be established between maceral composition and gas content, and that no strong correlations could be made between volatile matter, yield and coal rank or gas content. Since the boreholes were distal to the prominent geological structures in the coalfield Craig (2005) concluded that there are no structural controls on gas distribution, but that the depth of the coal seams appeared to have the greatest influence on gas contents.
Thomson et al. (2008, p. 7) proposed four distinct zones of gas layering in the lower part of the Hunter Coalfield. The layering with respect to gas composition was attributed to tectonic history and was regarded as being independent of coal seam depth. The distinct zones include Zone 1 which is the area near the surface characterised by high carbon dioxide concentrations originating from the oxidation of coal, with hardly any methane present. Zone 2 extends from below Zone 1 to about 400 m, where CSG consists mainly of biogenic methane. Zone 3 is a mixed gas zone with significant concentrations of both magmatic carbon dioxide and thermogenic methane, whereas gas in the deeper seams of Zone 4 contains mainly thermogenic methane and very little carbon dioxide (Thomson et al., 2008, p. 7). According to Thomson et al. (2008, p. 8–9), thermogenic methane was generated during the burial of coal seams in the Triassic and Jurassic, after which significant volumes of magmatic carbon dioxide were first introduced by igneous activity during the Jurassic and Cretaceous. Some of the trapped methane and carbon dioxide escaped as a result of basin uplift during the Late Cretaceous to Early Cenozoic, with uplift assisting the formation of biogenic methane through the reduction of magmatic carbon dioxide. Cenozoic volcanism then injected even more carbon dioxide into the basin, which was followed by continued generation of biogenic methane (Zone 2) and the oxidation of coal (Zone 1) to the present (Thomson et al., 2008, p. 8–9). This model was further developed by Thomson et al. (2014) who provided a rationale for the origin and timing of emplacement of the various coal seam gases in the western and eastern parts of the Sydney Basin.
In an investigation on the origin, distribution and controls on CSG in the Hunter Coalfield, Pinetown (2012, 2013) suggested that five CSG ‘compartments’ were present that have different gas attributes, structural features and local geology; one of which is located in the south, three in the central region, and one in the northern part of the coalfield. Within these compartments similar patterns exist for gas content and composition, coal petrology, and other reservoir characteristics, with boundaries defined by structures in some cases. In other cases compartment boundaries extend across structural boundaries. The compartments identified include the area south of the Hunter River Cross Fault, which is characterised on average by the highest gas contents (~9 m3/t), adsorption capacities (~23 m3/t), permeabilities (between about 100.0 and 1.0 mD) and vitrinite reflectances (0.56 to 1.15%) in the coalfield (Pinetown, 2012, p. 293). Of the three compartments located in the central region of the coalfield, with the Hunter-Mooki Thrust Fault and Muswellbrook Anticlines in the east, and the Mount Ogilvie Fault in the west forming the main boundaries, the eastern-most compartment contains predominantly methane, the central compartment is characterised by low gas contents, and the compartment west of the Mount Ogilvie Fault is considered to have intermediate gas contents (Pinetown, 2013, p. 145). In the north the compartment located to the west of the Aberdeen Thrust Fault is characterised by in situ gas contents of up to approximately 14 m3/t, and carbon dioxide as the dominant gas component (between ~60% and ~90%) from the surface to about 500 m depth (Pinetown, 2013, p. 148).
Following on from this, Pinetown (2014) used basin modelling to improve understandings of the origin and temporal evolution of CSG in the Hunter Coalfield. Burial history models show that coals in the south of the coalfield appear to have the greatest potential for thermogenic gas generation, and that those areas with low gas contents and decreased permeability have been uplifted more, and buried less, compared with areas that have high gas contents. According to Pinetown (2014, p. 422) it appears that present-day gas contents may partially reflect coal ranks and adsorption capacities, with late-stage biogenic gas generation replenishing methane volumes that were lost following uplift during the Late Cretaceous. Patterns of gas distribution in the Hunter Coalfield are controlled by a variety of factors, including the geological setting and burial history, which control migration of groundwater and therefore biogenic gas production (Pinetown, 2013, p. 147–150). Pinetown (2014, p. 423) suggests that the Hunter Coalfield is a compartmentalised CSG reservoir and that delineating compartments within the coalfield provides improved understanding of the CSG distribution, through which the implications for CSG exploration, coal mine safety and fugitive emissions from open-cut coal mines can be assessed.
Pinetown (2013) also studied gas distribution in the Hunter Coalfield as a natural analogue to assess the potential of carbon dioxide storage in coal. Using a simplistic approach a theoretical total storage capacity of about 9512 Mt of carbon dioxide is estimated for coals between 300 and 800 m depth, with an average coal permeability of 1.5 mD between these depths. According to Pinetown (2013, p. 155) the southern region of the coalfield shows the greatest potential for carbon dioxide storage given its high permeability, proximity to potential carbon dioxide sources, and thick sequence of coal seams, including deeper seams as possible storage targets. The actual available storage capacity, however, could be considerably less owing to factors such as high existing carbon dioxide concentrations in some areas, low permeability in many areas, the presence of national parks, and existing and proposed coal mines.
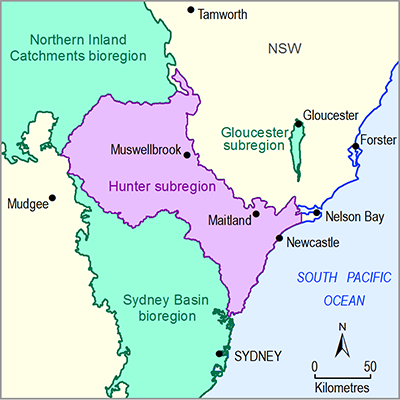
Product Finalisation date
- 1.2.1 Available coal and coal seam gas resources
- 1.2.2 Current activity and tenements
- 1.2.2.1 Coal
- 1.2.2.1.1 Awaba Colliery
- 1.2.2.1.2 Cumnock Mine
- 1.2.2.1.3 Mannering Colliery
- 1.2.2.1.4 Newstan
- 1.2.2.1.5 Ravensworth Underground Mine
- 1.2.2.1.6 Westside Coal Mine
- 1.2.2.1.7 Ashton Coal Mine
- 1.2.2.1.8 Austar Coal Mine
- 1.2.2.1.9 Bengalla Coal Mine
- 1.2.2.1.10 Bloomfield Coal Mine
- 1.2.2.1.11 Bulga Coal Complex
- 1.2.2.1.12 Chain Valley Colliery
- 1.2.2.1.13 Donaldson Coal Complex
- 1.2.2.1.14 Drayton Mine
- 1.2.2.1.15 Hunter Valley Operations
- 1.2.2.1.16 Integra
- 1.2.2.1.17 Liddell
- 1.2.2.1.18 Mandalong Mine
- 1.2.2.1.19 Mangoola Coal Mine
- 1.2.2.1.20 Moolarben
- 1.2.2.1.21 Mount Arthur Coal Mine Complex
- 1.2.2.1.22 Mount Owen Complex
- 1.2.2.1.23 Mount Thorley–Warkworth
- 1.2.2.1.24 Muswellbrook Coal Mine
- 1.2.2.1.25 Myuna Colliery
- 1.2.2.1.26 Ravensworth Complex
- 1.2.2.1.27 Rix's Creek Mine
- 1.2.2.1.28 Ulan
- 1.2.2.1.29 Wambo Mine Complex
- 1.2.2.1.30 West Wallsend Colliery
- 1.2.2.1.31 Wilpinjong Coal Mine
- 1.2.2.2 Coal seam gas
- References
- Datasets
- 1.2.2.1 Coal
- 1.2.3 Proposals and exploration
- 1.2.3.1 Coal
- 1.2.3.1.1 Bickham Coal Project
- 1.2.3.1.2 Bulga Coal Optimisation Project and Blakefield North Underground Mine
- 1.2.3.1.3 Bylong Coal Project
- 1.2.3.1.4 Chain Valley Modification 1
- 1.2.3.1.5 Dellworth Project
- 1.2.3.1.6 Doyles Creek
- 1.2.3.1.7 Drayton South Coal Project
- 1.2.3.1.8 Drayton Mine Extension Project
- 1.2.3.1.9 Ferndale Project
- 1.2.3.1.10 Kayuga Project
- 1.2.3.1.11 Mandalong Southern Extension
- 1.2.3.1.12 Mangoola Coal Exploration Program
- 1.2.3.1.13 Mannering Colliery Modification 2
- 1.2.3.1.14 Mitchells Flat Project
- 1.2.3.1.15 Monash Deposit
- 1.2.3.1.16 Mount Owen Continued Operations Project
- 1.2.3.1.17 Mount Penny Deposit
- 1.2.3.1.18 Mount Pleasant Mine Project
- 1.2.3.1.19 Spur Hill Project
- 1.2.3.1.20 Wallarah 2
- 1.2.3.1.21 West Muswellbrook Project
- 1.2.3.2 Coal seam gas
- References
- Datasets
- 1.2.3.1 Coal
- 1.2.4 Catalogue of potential resource developments
- Citation
- Acknowledgements
- Contributors to the Technical Programme
- About this technical product