1.1.4.2.1 Groundwater levels and flow paths
Alluvial aquifers
Regionally, groundwater gradients in the alluvial aquifers indicate flow from the east in a north-westerly to westerly direction away from the Namoi River (Parsons Brinckerhoff, 2011) and the groundwater flow systems within the major aquifers are generally local to intermediate in scale (CSIRO, 2007).
SWS (2011) developed a water level contour map for the wider Namoi catchment using average water levels for non-GAB bores. Watertable mapping indicates that groundwater flow in the Upper Namoi is generally south to north draining into the Lower Namoi through a bedrock constriction north of Narrabri (SWS, 2011; Barrett, 2012). Groundwater in the Lower Namoi generally flows from east to west.
Narrow geological constrictions along the Upper Namoi River valley have affected the deposition of the alluvial sediments, thereby restricting groundwater flow in the Upper Namoi Alluvium. Gins Leap north of Boggabri is the location of a major groundwater flow constriction due to aquifer geometry/extent (SWS, 2011).
The alluvial sequence is considered to be continuous over the Hunter-Mooki Fault which forms the boundary between underlying Mesozoic and Paleozoic strata so groundwater in the Narrabri and Gunnedah formations is likely to flow into the Namoi subregion from the east (SWS, 2011).
There is primarily downward flow of groundwater from the Narrabri to the Gunnedah formations in the eastern part of the subregion, however vertical hydraulic gradients are reversed at the western margin. In some locations which usually show potential for upward flow, heavy pumping of groundwater over the irrigation season reverses the flow potential (Parsons Brinckerhoff, 2011; Barrett, 2012).
Recent measurements (post-2000) of the watertable in the Narrabri Formation are shown in Figure 26. Groundwater mounds are developed about the Namoi River and its anabranch, Pian Creek, in the watertable in the Narrabri Formation. This indicates that these streams leak into the Narrabri Formation. The magnitude of this bed underflow leakage is estimated to be about 42 GL/year (CSIRO, 2007). Corroborating evidence is provided by Parsons et al. (2008) based on groundwater levels relative to surface water levels. Parsons et al. (2008) classify Pian Creek as a maximum losing stream and the Namoi River between Narrabri and Walgett as a medium to high losing stream.
In Figure 26, the Northern Namoi Paleochannel is depicted as a groundwater drain in the Narrabri Formation. The drain is generated by the large amount of groundwater pumping for irrigation mostly from the Gunnedah Formation over the Lower Namoi Valley but especially in the palaeochannel. While actual groundwater extraction in the Lower Namoi in the past three wet years has been below 50 GL/year (NSW Office of Water, 2013), the annual extraction limit for the Lower Namoi in 2013-2014 is set at 90.2 GL, and actual extraction over the past two decades has commonly exceeded 100 GL /year (Smithson, 2009). The groundwater pumping from the Gunnedah Formation induces significant downward leakage from the Narrabri Formation.
Figure 26 Watertable in the Narrabri Formation
Groundwater levels in alluvial aquifers in the Upper and Lower Namoi groundwater management areas have been monitored at 331 and 256 monitoring bore locations respectively, for the past 30 to 40 years (Smithson, 2009; Barrett, 2012). In some areas it can be difficult to distinguish between groundwater in the Narrabri and Gunnedah Formations at they can act as a single aquifer.
Groundwater in the alluvial aquifers is generally shallower at the eastern extent of the subregion, gradually becoming deeper from south to north in the Upper Namoi (Barrett, 2012). At Narrabri groundwater is around 4 to 12 m below ground level, becoming progressively deeper towards the west, and is around 25 to 34 m below ground level at Cryon. Long-term groundwater level declines at the western end of the valley, where usage is low, are most likely related to extraction higher in the valley limiting throughflow (Smithson, 2009).
In general, groundwater levels in the alluvial aquifers have responded to rainfall variability and associated variability in groundwater use. Groundwater levels have generally been falling since the late 1960s/early 1970s due to over-extraction, with water levels stabilising or recovering during wetter years when there has been reduced extraction. In 2006–07, many parts of the Namoi subregion experienced their lowest groundwater levels since monitoring commenced (Smithson, 2009; Burrell et al., 2011; Barrett, 2012).
The contrast between drier and wetter seasons affects groundwater levels as a result of differing rates of river recharge to the groundwater system, and changes to groundwater demand for irrigation. Where the surface water system is highly connected to the aquifer system, a wetter regime coincides with higher flows in the river, and an associated higher pressure head to promote recharge to the aquifer. The reduced demand for extractions would also be contributing to this shift in trend (Burrell et al., 2011). Such sites are also likely to recharge GAB aquifers via bed underflow leakage through the alluvium palaeochannel (Kellett et al., 2012). This effect is reduced in areas where the river is disconnected from the alluvial aquifers.
Hydrographs often show seasonal variations in groundwater levels in the alluvial aquifers in phase with groundwater extraction for irrigation. These variations are generally characterised by periods of falling and/or fluctuating groundwater levels from spring to autumn and an increase or stabilisation of groundwater levels during winter, in accordance with irrigation schedules. As there is little excess rainfall over evaporation even in the winter months, the observed seasonality is considered to be caused by changes in groundwater abstraction.
Great Artesian Basin
The first recorded bores tapping the GAB in the Namoi were noted in the 1890s. Historically bores were left uncapped, resulting in artesian water, where present, leaving the system and causing a decrease in pressure (SWS, 2011). The lowering of the potentiometric surface of the main GAB aquifers has caused it to fall below the ground surface in several areas and as a result flows from artesian water bores in those areas have ceased or diminished (CSIRO, 2012). Data from the NSW government database indicates groundwater levels near Pilliga, close to the Namoi recharge zone, fell from around 200 mAHD in 1915 to around 170 mAHD by the late 1980s (SWS, 2011).
Figure 27 is modified from the regional potentiometric surface developed for the Surat Basin as part of the Great Artesian Basin Water Resource Assessment (CSIRO, 2012). The surface was developed using all available groundwater data and shows that the groundwater in the GAB aquifers flows from south-east to west and north-west. The watertable lies in the main GAB aquifer in New South Wales, the Pilliga Sandstone. It then passes into thin bands of Keelindi and Drildool Beds and then into undifferentiated Rolling Downs Group to the west, outside of the Namoi subregion (CSIRO, 2012). In contrast to the Narrabri Formation groundwater contours in Figure 26, the potentiometric surface in the uppermost GAB aquifer (Figure 27) does not generally show any obvious groundwater/surface water interaction along the Namoi River and its anabranch, Pian Creek (the potentiometric surface is not distorted/impacted near these surface water features). One possible exception is the area just downstream of Narrabri at the confluence with Bohena Creek.
Figure 27 Potentiometric surface of groundwater in the uppermost Great Artesian Basin aquifer
Source data: modified from CSIRO (2012)
Except for a small area at Pilliga, heads in the regional GAB watertable (Figure 27) are above heads in the Narrabri Formation (Figure 26). This is in marked contrast to the situation in the early 1990s where Williams (1997) showed the head in the alluvium as lying above the regional GAB watertable except for a small area at Narrabri West. This means that in approximately 20 years there has been a reversal in the head differential between the two flow systems due to groundwater pumping for irrigation. The dotted area at Pilliga in Figure 26 probably indicates a zone where groundwater in the alluvium is perched above the regional GAB watertable aquifer since it is likely that the saprolite aquitard on the Griman Creek Formation is preserved there.
Gunnedah Basin
There is very little published information relating to the hydraulic properties of the Gunnedah Basin hard rock aquifers, so groundwater levels and flow paths are largely unknown.
1.1.4.2.2 Groundwater quality
The collection of groundwater quality data in the Namoi subregion has tended to be infrequent and irregular, and undertaken by a range of agencies including government departments, research organisations and consultants. These studies have tended to be very site specific and short-term, and show a large amount of spatial variability. Documentation relating to sampling methodologies and instruments is often lacking and the limited transfer of data into central databases makes the interpretation of historical chemical data difficult (Kelly et al., 2007). SWS (2011) note that for a catchment the size of the Namoi, there is very little groundwater quality data available from the NSW Office of Water Pinneena database. According to the database, water quality records have been collected from 1991 onwards, however, there are insufficient water quality data to establish the variation in major ion chemistry over time. Additionally, the depth from which samples have been taken can be difficult to determine because in locations with nested piezometers installed the database does not distinguish from which depth the measurement was taken (SWS, 2011).
Groundwater salinity is the main measure of groundwater quality that has been collected in the Namoi subregion because this has been a prominent issue in several areas due to shallow watertables and an increase in irrigation water salinity in some areas (Kelly et al., 2007; Timms et al., 2010). However, there are limited long-term and/or periodic salinity monitoring records in the NSW Office of Water database and historic single point values are often grouped into numerical classes or are descriptive (e.g. fresh, brackish, salty, etc.) (SWS, 2011).
Figure 28 shows suitability of groundwater in the alluvium (based on salinity) for various purposes (Green et al., 2011). Groundwater is generally less saline in the Upper Namoi area, increasing away from the Namoi River and downstream. Previous studies also indicate that the alluvial aquifers are often fresher at depth (Lavitt, 1999; McLean, 2003; Parsons Brinckerhoff, 2011).
An increase in groundwater salinity, possibly due to increased saline drainage through the alluvium, has been reported in some irrigation areas of the Lower Namoi since the 1990s. Groundwater salinity appears to have increased during the 1990s at some sites in the Upper Namoi as well, possibly limiting beneficial use (Timms et al., 2010).
Figure 28 Alluvial groundwater quality and suitability in the Namoi area
Source: Figure 13 in Green et al. (2011)
As discussed above there are very few groundwater chemistry data in the NSW government database, however a number of research projects have analysed groundwater chemistry in the Namoi subregion, as summarised below.
Upper Namoi
Groundwater salinity across the Upper Namoi Alluvium is generally less than 1500 μs/cm, with areas of higher salinity up to 7000 μs/cm (Barrett, 2012).
Lavitt (1999) found that groundwater in the shallow alluvium (Narrabri Formation) of the Mooki catchment had a highly variable composition but was more similar to river water than the deeper (Gunnedah formation) alluvial aquifers. This implies a limited vertical connection within the alluvium under natural conditions in this area. Timms and Acworth (2002) found a similarly disconnected alluvial system in the upper Mooki catchment, with a low rate of vertical leakage between the shallow and deeper alluvial layers.
Lavitt (1999) found samples from the deeper alluvial units were similar to the surrounding hard rock units, suggesting hydraulic connection between the deeper alluvium and shallow hard rock aquifers. However, it may also indicate the source rock of the deeper alluvium is similar to the hard rock aquifers, or that the water in the deeper alluvium is older and more chemically evolved than in the shallow alluvium.
Similarly, Timms and Acworth (2002) found that the water chemistry of the deeper Mooki alluvium was similar to that of the Liverpool Ranges Volcanics and Gunnedah Basin sediments. Similar results were found in the Coxs Creek catchment.
The results of a recent hydrogeochemical sampling and characterisation project of alluvial groundwater in the Upper Namoi (Parsons Brinckerhoff, 2011) indicated that:
- The Narrabri Formation is dominated by sodium and chloride. Major ion chemistry in the Gunnedah Formation is spatially variable, indicating the aquifer is laterally discontinuous with zones of differing salinity and major ion composition.
- Groundwater quality has deteriorated in some bores in the Narrabri Formation (no longer suitable for stock) and Gunnedah Formation (from irrigation to stock).
- Some bores in the Gunnedah Formation showed a long-term increasing trend in salinity, which is attributed to either downward leakage of saline water where aquitards are thin or absent, or to leakage of saline water from clay aquitards as a result of depressurisation where persistent abstraction has occurred.
- Processes influencing the major ion composition of groundwater include mixing, ion exchange, weathering of silicate minerals and clays and dissolution and precipitation of minerals such as carbonates and gypsum.
- A change in water type has occurred in some Gunnedah Formation bores where salinity is increasing.
Lower Namoi
Groundwater salinity generally increases away from the main recharge areas in the east (Figure 28), and from the mean Namoi River EC of 560 μS/cm to over 30,000 μS/cm in the Narrabri Formation (Barrett et al., 2006, as cited in Parsons Brinckerhoff, 2011).
McLean (2003) analysed changes in groundwater salinity between the mid 1980s and 1999 and concluded that the salinity of groundwater in the eastern part of the Lower Namoi had increased by 100 μS/cm over the last 30 years, and in the western portion of the aquifer by several thousand μS/cm. These increases have been attributed to changes in direction of potential flow paths caused by pumping practices (Kelly et al., 2007). The changes in water quality due to pumping were localised and showed no general trends (McLean, 2003).
The results from a recent hydrogeochemical sampling and characterisation project of alluvial groundwater in the Lower Namoi (Parsons Brinckerhoff, 2011) indicated that:
- Groundwater levels have declined by 1.5 to 4 m since the late 1970s.
- Major ion chemistry in all aquifers is dominated by sodium and chloride.
- The beneficial use of groundwater has deteriorated at some bores in the Narrabri Formation (no longer suitable for stock), Gunnedah Formation (no longer suitable for some crops including cotton) and Cubbaroo Formation (no longer suitable for some crops including cotton) since monitoring began.
- Some Gunnedah Formation bores showed a long-term increasing trend in salinity, which was attributed to vertical leakage of saline water from the upper aquifer and saline intrusion of pore waters. One Narrabri Formation bore and one Cubbaroo Formation bore also showed a long-term increasing trend in salinity.
- Processes influencing the major ion composition of groundwater include mixing, ion exchange, reverse ion exchange, and dissolution and precipitation of minerals such as carbonates and gypsum.
- There were no long-term changes in water type identified.
Great Artesian Basin
Groundwater in the Pilliga Sandstone aquifers in the Namoi subregion is generally of good to fair quality and suitable for stock and domestic use. Salinity in the most widely-used aquifers is low but becomes more variable with depth. Groundwater salinity generally increases from the recharge areas along the groundwater flow path towards the north and west (GABCC, 2010). The increasing salinity trend may be a consequence of soluble ions leaching from overlying or underlying formations with marine depositional histories, the mixing of dilute recharge waters with saline waters present within deeper parts of the GAB and the dissolution or weathering of evaporates, carbonate minerals or incongruent dissolution of feldspars, micas or clay minerals along the flow path (Herczeg et al., 1991).
Following infiltration in the recharge zones, groundwater in the GAB aquifers tends to evolve from mixed cation-bicarbonate-chloride to sodium-bicarbonate-chloride dominant groundwater as a result of carbon dioxide production, carbonate dissolution, ion exchange and aluminosilicate weathering (Herczeg et al., 1991; Radke et al., 2000).
GAB groundwater tends to have high concentrations of sodium. This renders the water unusable for irrigation in most places due to high sodium absorption ratios in both the soil and applied groundwater. However, further north in the North Star/Yallaroi area, a combination of lower sodium groundwater and sandy soils allows irrigation to occur in the recharge areas on the eastern margin of the Basin. Sodium concentrations as low as 25 mg/L occur in the recharge areas, increasing progressively to over 1000 mg/L in the far west (GABCC, 2010).
Alkalinity (as bicarbonate) is the dominant anion and chloride concentrations are very low in the eastern recharge areas and the Coonamble Embayment (<50 mg/L) (GABCC, 2010).
Studies of stable isotope ratios of oxygen and deuterium (δ18O and δ2H) show that GAB groundwater is isotopically heavier (below the meteoric water line) than rainfall, indicating groundwater has probably undergone evaporation or other processes. Plots of samples from specific regions, such as the recharge areas, central parts and discharge areas of the GAB provide different patterns. All δ18O and δ2H plots indicate that the artesian groundwater is meteoric in origin (CSIRO, 2012).
Chlorine-36 data for the New South Wales portion of the GAB indicate flow systems increasing in age along the inferred hydraulic gradient, with indicative groundwater ages ranging from less than 5000 years, within the highland areas and river alluvial valleys in the recharge zone, to greater than 200,000 years, in the western part of the Coonamble Embayment (Radke et al., 2000). The inferred horizontal flow rates are 4 m/year in the alluvial fans that abut the Great Dividing Range, decreasing to less than 1 m/year as groundwater traverses towards the plains (Herczeg, 2008).
Gunnedah Basin
Groundwater chemistry data from Gunnedah Basin sedimentary sequences is limited to localised investigations around prospective CSG operations. From the limited published data available, most groundwater samples from the non-coal seam formations in the Gunnedah Basin are fresh to slightly brackish. In contrast, groundwater samples from the Hoskissons Coal Member are predominantly brackish. An increasing salinity trend was observed in the following order:
Narrabri/Gunnedah < Pilliga Sandstone < Napperby/Digby Formation < Black Jack Group < Hoskissons Coal (Golder Associates, 2010, 2011).
Major ion analysis of a very limited number of groundwater samples in the Gunnedah Basin shows groundwater from the Hoskissons Coal Member generally has high concentrations of sodium and bicarbonate, with the lowest concentrations of sulfate and chloride. Groundwater from other formations, including the Napperby and Digby formations, is characterised by a range of water types reflecting the heterogeneity of the sedimentary environment (Golder Associates, 2010, 2011).
Eastern Star Gas (now Santos) published typical values for produced water from their target coal seams in the Gunnedah Basin (Eastern Star Gas, 2006). These values are summarised in Table 7. Typical of CSG co-produced water, it is high in TDS and is of sodium-bicarbonate water type.
Santos has also published groundwater quality data for the Hoskissons Coal Member and the wider Gunnedah Basin units as shown in Table 7. Similarly to the Eastern Star Gas data, the groundwater in the coal seam is dominated by sodium and bicarbonate, although the absolute values for the Hoskissons seam are lower than those provided by Eastern Star Gas. These results are typical of the water composition in coal seams exploited for gas (low in calcium, magnesium and sulfate and dominated by sodium, bicarbonate, and often chloride where there are marine associations) (Van Voast, 2003). The water quality of the Gunnedah Basin units is noticeably different to the coal seams and generally better quality (Golder Associates, 2010).
Table 7 Indicative groundwater quality data from coal seams in the Gunnedah Basin
Source data:1Eastern Star Gas (2006). Sample collected 25 July 2006; 2Golder Associates (2010). Mean groundwater values from Santos’ Longlea and Georges Island wells; 3Golder Associates (2010). Mean groundwater values from 15 to 17 samples
1.1.4.2.3 Groundwater recharge
Recharge to the alluvial aquifer system mainly occurs in the east via several mechanisms including from the Namoi River and its tributaries, especially during major flooding events, rainfall infiltration, irrigation, through flow from surrounding aquifers and catchments, and on-farm water losses. Recharge can also occur via upward leakage of groundwater from the underlying aquifers (Salotti, 1997; CSIRO, 2007; Parsons Brinckerhoff, 2011).
Rainfall recharge
Rainfall recharge in the western part of the Namoi subregion is considered to be low. The Lower Namoi catchment groundwater model indicates that diffuse recharge from rainfall is a minor recharge source (Merrick, 2001a). Timms et al. (2012) found that the watertable at the western extent of the subregion near Walgett occurs at 20 m depth and that there is generally no significant groundwater level response to large rainfall events, indicating little diffuse rainfall recharge. The lack of groundwater level response was expected given the large water holding capacity of the surface clays and the low hydraulic conductivity limiting vertical groundwater flow. High frequency groundwater level data indicated that recharge is <0.6% of annual rainfall during very wet years and is limited to localised areas of the plains. The mean long-term rainfall recharge rate could be as low as 0.01%. Considering the uncertainties involved, recharge is considered very small or negligible (Timms et al., 2012). This is in contrast to rapid groundwater level response to rainfall detected in the Upper Namoi catchment, resulting in elevated groundwater levels which then dissipate during dry periods (Timms et al., 2001; Timms and Acworth, 2005).
River recharge
The streams on the alluvial plain flow across the top of the Narrabri Formation. At the eastern and western margins of the plain, the rivers are in direct hydraulic contact with the watertable. An unsaturated zone develops between these points where the watertable falls below the streams, and surface water recharges the underlying aquifer while streamflow persists.
Irrigation recharge
Groundwater recharge as a result of irrigation may represent a significant component of recharge in some areas and be an important mechanism for rising groundwater and salinity, especially in cracking clay soils, however estimates of groundwater recharge from irrigation vary considerably.
Lysimeter studies undertaken near Narrabri showed drainage ranged from approximately 30 to 50 mm/year during the cotton irrigation season, was 23 mm under fallow and negligible under wheat. Peak drainage rates occurred 25 hours after irrigation at over 3 mm/day then declined exponentially over about a week to 0.5 mm/day. The results from the lysimeter were compared to estimates of deep drainage by chloride mass balance, with the latter underestimating drainage by 55% (Ringrose-Voase and Nadelko, 2011).
Seasonal drainage from the root zone appeared to recharge the watertable at 16 m depth within weeks at a rate of 0 to 0.7 mm/day, however the result remains tentative (Ringrose-Voase and Nadelko, 2011). Under furrow irrigation Timms and Acworth (2002) found shallow groundwater levels increased by up to 3 m during the irrigation season and then declined rapidly, not showing a general rising trend. Kelly and Acworth (2005) used resistivity imaging to show that deep drainage occurs in isolated zones and that under furrow irrigation water drains beyond 5 m within 24 hours. More work is needed to understand if this water migrates laterally, returns to the surface water flows or moves downwards to recharge deeper aquifers.
Recharge from overlying and underlying aquifers
The Gunnedah Formation is mainly recharged via infiltration from the overlying Narrabri Formation. In general, there is a downward movement of groundwater from the Narrabri Formation to the Gunnedah Formation in the east, while at the western margin the direction is reversed (CSIRO, 2007). The aquifers of the Cubbaroo and Gunnedah Formations are also recharged in part by upward leakage from the GAB (Ransley et al., 2012a).
The Great Artesian Basin recharge
Recharge of the GAB aquifers takes place chiefly along the south and eastern fringe of the GAB, in an area known as the ‘intake beds’, and is derived from rainfall and streamflow. The location of the GAB intake beds in the Namoi is shown in Figure 29. Localised recharge can be relatively fast and effective depending on the depth and configuration of the regional watertable and the hydraulic characteristics of the overlying material (CSIRO, 2012).
Figure 29 Groundwater recharge areas for the Great Artesian Basin in New South Wales
The recharge rates for the Pilliga Sandstone intake beds are difficult to determine and there have been wide ranging estimates over nearly two orders of magnitude. The most recent assessment of recharge to the wider Pilliga Sandstone was undertaken as part of the GAB Water Resource Assessment, using chloride concentrations in groundwater and rainfall (CSIRO, 2012). The recharge flux in the entire Pilliga Sandstone intake beds was calculated as 84,000 ML/year. This estimate is compatible with the Queensland data and is also reasonably close to the recharge estimate made by DPI (2009) for the New South Wales GAB water sharing plan of 61,400 ML/year (less than 2% of rainfall) (Kellett et al., 2012).
1.1.4.2.4 Groundwater discharge
Groundwater in the alluvial aquifers can discharge to the Namoi River and other creeks or tributaries where the rivers are connected and gaining (short river reaches in the Upper Namoi River). The alluvial aquifers will also discharge groundwater vertically where hydraulic properties permit, into the deeper alluvial units, the GAB or the Gunnedah basin. Groundwater in the far west of the subregion moves slowly through the alluvial aquifers to the south-west out of the region and cannot discharge to the surface as the Namoi River bed is located at least 10 m above the watertable (Timms et al., 2012).
1.1.4.2.5 Aquifer connectivity
Alluvial aquifers
The greatest aquifer interaction in the Namoi subregion occurs through horizontal and vertical connections within and between alluvial aquifers. The alluvial areas are heterogeneous deposits and although they are commonly subdivided into the Narrabri and Gunnedah formations, the actual boundary depth is difficult to determine from geological bore logs (SWS, 2011).
Aquifer connectivity can be assessed by hydrograph analysis of nested bores screened in different aquifers. The more similar the hydrographs between the bores, the more likely that the aquifers are in good hydraulic connection. There are a large number of nested bores in the Namoi subregion that could be used to assess connectivity between alluvial aquifers, however studies to date have only selected a few bores for this analysis. Consequently, while leakage between the Narrabri and Gunnedah formations has been shown to occur in parts of the Namoi subregion, the extent of this connectivity is unknown.
Connectivity has also been demonstrated by extraction from the Gunnedah Formation inducing leakage from the overlying Narrabri Formation, in some cases resulting in dewatering of the upper aquifer (Barrett, 2012). The depth at which the alluvial systems react to deep abstractions varies over short distances (SWS, 2011).
The Great Artesian Basin aquifers
Close to the margins of the GAB, palaeochannels of overlying alluvium can be incised into aquifer units such as the Pilliga Sandstone, resulting in upward leakage where the palaeochannel has eroded the saprolite. Such connectivity is poorly known but is considered to be highly variable across the region (Ransley et al., 2012b) and may be significant where these palaeovalleys occur. The contribution of the GAB aquifers to the alluvium in the water balance of the Lower Namoi model has previously been calculated as approximately 3.8 m3/d/km2 (Herczeg, 2008, as cited in SWS, 2011). Estimations of upward leakage from the GAB aquifer into alluvial palaeochannels suggest this volume to be approximately 1450 ML/year for 400 km2 of palaeochannel (similar to 3.8 m3/d/km2). Where palaeochannels are absent, the GAB rocks are overlain by a layer of saprolite which greatly limits connectivity between the GAB and the overlying alluvial aquifers. Water pressures in the GAB have fallen significantly since the aquifers were first tapped, and the potential for upward movement of water into the alluvium will have reduced as the pressure gradient has fallen.
A preliminary desktop assessment of the potential for hydraulic connectivity between the GAB with the overlying alluvium and underlying basins was undertaken as part of the Great Artesian Basin Water Resource Assessment (CSIRO, 2012). Connectivity can occur where aquifers and leaky aquitards are juxtaposed below and above the base of the GAB. Figure 30 shows that the hydraulic connectivity between the GAB and underlying Gunnedah Basin appears to be variable but limited across the Namoi subregion (CSIRO, 2012). There may be some connectivity in the northern part of the subregion between the GAB and the underlying Gunnedah Basin, as indicated in Figure 30.
Source data: derived from data described in CSIRO (2012)
Many of the active and planned coal mines and CSG operations have completed site specific numerical groundwater models (e.g. RCA Australia, 2005; Golder Associates, 2010) which have predicted only small changes in water levels outside the targeted areas, indicating they assume limited connectivity in these locations.
Gunnedah Basin aquifers
The degree of connectivity between the alluvium, GAB and Gunnedah Basin aquifers is a key component for determining any impacts on water resources within the subregion due to coal mining or CSG extraction. However, information and data on connectivity are very limited and represent a significant knowledge gap. Comprehensively defining the connection between different geological basins and the role of large-scale development on groundwater in the Namoi subregion would require closing some of the following knowledge gaps:
- Quantifying the hydraulic connection between the shallow alluvial aquifers, the GAB and underlying geological basins. While potential ‘windows’ of connectivity between basins and between surface and groundwater have been identified, the rates of groundwater exchange remain unknown.
- The controlling mechanisms for vertical leakage (cross-formational flow) for the multiple layers of aquifers and aquitards present in the Namoi subregion. Understanding these mechanisms is critical for determining the effect of depressurisation proposed for CSG development in the region.
- The hydraulic properties of aquitards and response to changes in groundwater pressure within adjacent aquifers. Where several layers of aquifers and aquitards are present, pressure changes caused by groundwater extraction will propagate at various rates in various directions, depending on the physical properties unique to each aquifer and aquitard layer (CSIRO, 2012).
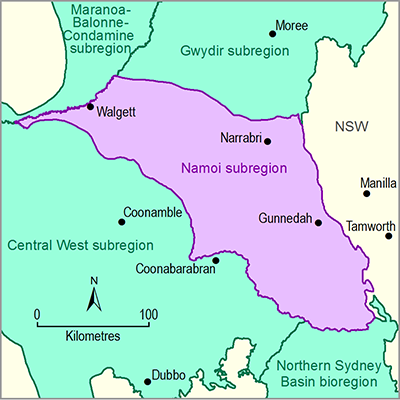