The groundwater flow systems of the Gippsland Basin are generally not in a steady state (Nicol, 2010; Underschults et al., 2003; Walker and Mollica, 1990). Groundwater extraction for oil and gas production, coal mining, irrigation and water supply, as well as land use change including land clearing, establishment of irrigation agriculture and afforestation for forest production have significantly altered the recharge and discharge characteristics of the groundwater flow systems of the Gippsland Basin over the last four decades.
The flow systems of the Tarwin sub-basin and the Moe Swamp sub-basin are distinct from each other and those of the Gippsland Basin as a result of structural elements that impede hydraulic connection. This is discussed further in Section 1.1.4. 3.2, Section 1.1.4.3 .3 and Section 1.1.4. 3.4.
1.1.4.3.1 Gippsland Basin
Within the Latrobe Valley and Lake Wellington depressions, vertical head gradients are downward in the west, implying potential for downward flow; and upwards in the east, implying potential for groundwater flow from deeper to shallower units. The upward head gradients are locally large – especially in the area around the Gippsland Lakes where some groundwater is artesian (Hofmann and Cartwright, 2013). Groundwater modelling studies within the Gippsland Basin have suggested that up to one-third of groundwater extracted from the coal-bearing aquifers (the lower aquifer system and the middle aquifer system) within the Latrobe Valley Depression originates from storage in adjacent aquitards, indicating that inter-aquifer leakage may be a significant source of recharge to these aquifers (Schaeffer, 2008). Hofmann and Cartwright (2013) also found evidence of leakage and inter-aquifer connectivity across the Gippsland Basin in their study of the hydrogeochemistry of the Gippsland Basin. Hydrographs for the various aquifer systems show significant contrasts in trends between the deeper confined aquifers of the lower aquifer system and those of the shallower aquifers.
Figure 24, Figure 25 and Figure 26 present modelled groundwater level surfaces for the Gippsland Basin bioregion. The surfaces are based on preliminary modelling and may be subject to change as modelling progresses for the bioregional assessment. They are presented here to indicate the general direction of groundwater flow in these aquifer systems as well as, in general, the changes in groundwater level that have occurred over the last three decades. The trends visible in these maps are discussed further in the section below with the aid of hydrographs.
Data: Victorian Government (Dataset 2)
Data: Victorian Government (Dataset 2)
Data: Victorian Government (Dataset 2)
Bedrock aquifer system (Cretaceous and Ordovician to Carboniferous): Strzelecki Group and Paleozoic basement sediments and intrusives
The Cretaceous age Strzelecki Group and the Paleozoic rocks are often referred to as groundwater basement due to their low matrix permeability. Groundwater flow does occur in local to intermediate flow systems, dominated by fracture flow. Some localised matrix flow may also occur in the sandier facies and coal seams of the Strzelecki Groups rocks (e.g. Harrison et al. (2012) reported thermal anomalies within the Strzelecki Group that are attributed to groundwater flow in major coal seams within the Strzelecki Group). Groundwater flow in fracture networks is thought to be limited to the upper 50 to 100 m of bedrock and the aquifer system is considered to undergo relatively minor regional throughflow (Nicol, 2010). Groundwater is likely to be recharged locally, transmitted rapidly through fracture networks and ultimately contributes to stream discharge (Nicol, 2010). Previous work has not included detailed study of the hydraulic properties and groundwater flow dynamics of the bedrock aquifer system within the Gippsland Basin bioregion. Should the Strzelecki Formation be found prospective for unconventional gas resources, it is likely that further characterisation of the aquifers and flow systems of the Strzelecki Group will be required to inform both baseline characteristics as well as predictions of the possible changes that may be caused by unconventional gas development. Figure 27 shows a typical hydrograph (bore 144469) for the fractured rock aquifer system. It shows that groundwater levels increase and decline seasonally but generally fluctuate around a central value indicating local flow systems are dominant and that depletion via drainage of fractures either by pumping or to surface water systems is seasonally replenished by rainfall recharge. This is also evident in the increasing trend post 2010 reflecting a return towards average rainfall conditions following the millennium drought.
Lower aquifer system (Eocene to Oligocene): Latrobe Group, basal coal units of the Morwell Formation sediments and volcanics
Recharge to the Latrobe Group aquifer system occurs from rainfall infiltration and stream leakage in areas of outcrop and shallow subcrop. The aquifer system outcrops and subcrops around the basin margins in the west and the north to as far east as about Boisdale, and subcrops beneath Quaternary sediments on the crest of the Baragwanath Anticline and on local structural highs such as the Loy Yang Dome and Hedley Dome (Walker and Mollica, 1990).
Groundwater flow is generally from the recharge areas in the west, towards the offshore to the east and south-east. Natural groundwater discharge is thought to occur offshore where the Latrobe Group sediments are exposed on the continental shelf (Walker and Mollica, 1990). Flow directions are complicated by features such as the Baragwanath Anticline, Rosedale Fault and the associated Rosedale Monocline, which direct flow from the west in an easterly direction until the anticline plunges to sufficient depth to allow flow towards the offshore basin (Nicol, 2010). Groundwater discharge from the Latrobe Group aquifer in the onshore part of the basin occurs at the Dutson Springs on the Baragwanath anticline and around the Hedley Dome near Gelliondale (Thompson and Walker, 1982).
Groundwater flow has been considerably altered since the 1960s as a result of dewatering operations at the Morwell and Loy Yang open-cut mines. Groundwater now flows radially towards the open-cut mine. Groundwater extraction from the Latrobe Group aquifer for the production of oil and gas exceeds estimated annual recharge, causing a 1 to 1.5 m/year decline in groundwater level in the Latrobe Group aquifer within the Latrobe Valley, Lake Wellington Depression and Seaspray Depression since the 1960s to 1970s (Nicol, 2010; Underschultz et al., 2006; Walker and Mollica, 1990). The Traralgon Formation typically shows the greatest and most consistent rates of groundwater level decline in the Gippsland Basin (Nicol, 2010). Groundwater levels in the Latrobe Group aquifer have fallen by approximately 20 m in bores around the coal pits between 1980 and 2010. The cone of depression was located around Morwell in the 1990s and spread to the west towards Traralgon, where head values dropped by 68 m between 1990 and 2008. The groundwater divide around the Balook Block and Baragwanath Anticline shifted to the west as a consequence of the decreasing water levels in the area around the coal mines (Hofmann and Cartwright, 2013).
Thompson and Walker (1982) placed the freshwater-seawater interface within the Latrobe Group aquifer in the Seaspray Depression at about 30 km offshore. A more recent assessment of the location of the freshwater interface is not available.
Figure 28 shows two representative hydrographs for the lower aquifer system within the Gippsland Basin. Bore 67441 is representative of the region of the aquifer north and east of the Baragwanath Anticline, where the aquifer is covered by a thick sequence of sediments of the overlying stratigraphic sequence. The trend evident in the hydrograph is dominated by drawdown from offshore oil and gas production and onshore coal mine floor dewatering. It shows a strongly confined response reflecting significant drawdown and limited recharge. Bore 110724 is located to the south of the Baragwanath Anticline, where the Latrobe Group is closer to the surface, in many areas subcropping the Haunted Hills Gravel, and where it is used intensively for onshore water supply. The hydrograph for bore 110724 shows the effects of offshore oil and gas extraction. In the general decline trend, however, overprinting the drawdown associated with those abstractions are the seasonal abstractions from onshore water supply bores which suggest elastic storage depletion and recovery corresponding to irrigation water demand seasonality. The trend decline may also result in part from these water demands.
Middle aquifer system (Oligocene to Miocene): Latrobe Valley Group and Seaspray Group sediments
Recharge to the marine sediments of the Seaspray Group occurs via rainfall infiltration where these rocks outcrop or are in shallow subcrop. Minor recharge may also occur laterally from groundwater flow from the Balook Formation and the Latrobe Valley Group aquifers (Walker and Mollica, 1990). Groundwater flow within the marine rocks is towards the offshore part of the Gippsland Basin, however, the marine sediments are poor aquifers and aquitards and in the main, act to deflect groundwater upwards as it flows laterally from the non-marine stratigraphically equivalent sediments.
The marginal marine Balook Formation and the non-marine Latrobe Valley Group and Boisdale Formation are all hydraulically connected. Recharge to the system is around the margins of the Gippsland Basin from rainfall infiltration and river leakage. Vertical recharge via leakage from overlying aquifers is also likely to be an important source of recharge, particularly in areas of the aquifer system with large drawdowns caused by groundwater extraction (e.g. Latrobe Valley Depression) (Walker and Mollica, 1990). Recharge is also thought to occur where the sediments subcrop the Haunted Hills Gravel. In particular, this mechanism may be significant for recharge to the Boisdale Formation where the Boisdale Formation subcrop is traversed by the paleochannels and present-day courses of the Latrobe, Thomson, Macalister and Avon rivers (HydroTechnology, 1994; Walker and Mollica, 1990). In the area north and north-west of Sale, the Wurruk Sand Member of the Boisdale Formation subcrops the Haunted Hills Gravel and recent alluvium, providing a very good pathway for recharge from the surficial aquifers to the Boisdale Formation and is considered a major recharge area (HydroTechnology, 1994).
Groundwater flow in the Latrobe Valley Group sediments in both the Latrobe Valley and Lake Wellington depressions is essentially from west to east. It is thought that the flow passes into the Balook Formation and is largely deflected upwards to the Boisdale Formation by the less permeable, marine units of the Seaspray Group. The Latrobe Valley Group aquifers are generally deeply buried and not intensively targeted for groundwater extraction, however there is some stock and domestic use at the western end of the Seaspray Depression and in the Latrobe Valley. There is also dewatering of the aquifers of the Latrobe Valley Group within the Latrobe Valley Depression for coal mining and extraction of groundwater from these aquifers for power station cooling. These extractions locally influence flow directions (Nicol, 2010).
Movement of groundwater in the Boisdale Formation is west to east in the Latrobe Valley Depression and elsewhere it is north to south towards the offshore part of the basin. The Boisdale Formation passes laterally into the Jemmys Point Formation in the east (which acts as an aquitard) restricting lateral flow and potentially diverting groundwater upwards (Walker and Mollica, 1990). Hydraulic gradients from the Boisdale Formation to Lake Wellington suggest groundwater discharges from the Boisdale Formation to the lake (Hofmann and Cartwright, 2013; Walker and Mollica, 1990).
The Boisdale Formation is used over a large part of the basin to depths of 50 to 100 m for stock and domestic supplies and, in the area around Sale, for irrigation supplies. It is also used for town water supply at Sale (Walker and Mollica, 1990). Groundwater levels in the Boisdale aquifer system have been in gradual decline as a result of overallocated groundwater across the aquifer (Gippsland Water, 2012). Groundwater levels in the Boisdale aquifer near Sale declined by 5 to 10 m between 1990 and 2008 (Hofmann and Cartwright, 2013). Aquifer depressurisation for coal mining and oil and gas development is generally not considered to have affected the groundwater levels of the Boisdale Formation (Nicol, 2010).
Figure 29 shows five hydrographs within the middle aquifer system: bores 52753, 65762, 67442, 86466 and 92175. These bores are all screened within the Boisdale Formation aquifer.
Upper aquifer system (Pleistocene to Holocene): Sale Group sediments
The Haunted Hills Gravel conformably overlies most of the Gippsland Basin. Recharge is via rainfall infiltration and river leakage. There is significant recharge to underlying aquifers of the Gippsland Basin via the Haunted Hills Gravel.
Recharge to the Quaternary dune and sand aquifers occurs via local rainfall infiltration. Recharge to the Quaternary alluvium of the Mitchell, Avon, Thomson, Macalister and Latrobe rivers is by river leakage, flood recharge and rainfall infiltration. In some areas, Quaternary aquifers discharge to the rivers, and the pattern of recharge and discharge relationships between the Quaternary aquifers and rivers is in many areas seasonal (Walker and Mollica, 1990). This will be discussed further in Section 1.1 .6.
Aquifer depressurisation for coal mining and oil and gas development is not considered to have affected the groundwater levels of the Quaternary aquifers and the aquifers of the Haunted Hills Gravel (Nicol, 2010).
Figure 30 shows two representative hydrographs (bores 80762 and 105484) for the upper aquifer system which indicates there is no apparent influence of pumping from the underlying aquifers.
Data: Victorian Government (Dataset 3)
Data: Victorian Government (Dataset 3), Bureau of Meteorology (Dataset 4)
Data: Victorian Government (Dataset 3), Bureau of Meteorology (Dataset 4)
Data: Victorian Government (Dataset 3), Bureau of Meteorology (Dataset 4)
1.1.4.3.2 Tarwin sub-basin
The groundwater flow systems of the Tarwin sub-basin are isolated from those of the Gippsland Basin due to the structural controls on the basin. The Thorpdale Volcanics form the primary aquifer in the Tarwin sub-basin, and are considered to be in direct hydraulic connection with the overlying Haunted Hills Gravel, forming a largely unconfined aquifer (Nicol, 2010). Most groundwater flow is from the west and north-west to the south-east, where the Koorooman Fault largely forces groundwater to discharge upward into the Tarwin River as baseflow. Recharge largely occurs via rainfall infiltration to outcropping Haunted Hills Gravel, Thorpdale Volcanics, and Quaternary units (Nicol, 2010). Recharge may also occur as leakage from the Tarwin and Powlett rivers and their tributaries (Walker and Mollica, 1990). The Haunted Hills Gravel is considered to be in direct hydraulic connection with the Thorpdale Volcanics in the Tarwin sub-basin.
Aquifer depressurisation for coal mining and oil and gas development in the Gippsland Basin is not considered to have affected groundwater levels in the Tarwin sub-basin (Nicol, 2010). The majority of the groundwater extracted in the Tarwin sub-basin is from the volcanic aquifers. Figure 28 shows a representative hydrograph for the groundwater levels in the Tarwin sub-basin (bore 75399). Seasonal pumping influences are evident as well as the absence of trend. This likely reflects the limited storage of the volcanic aquifer and the relatively localised flow systems allowing for rapid drawdown and recovery via rainfall recharge.
1.1.4.3.3 Moe Swamp sub-basin
The sand, gravel and fractured basalt of the Childers Formation, Yarragon Formation and Thorpdale Volcanics are confined with artesian conditions occurring in the southern and central part of the basin. Low permeability layers are present within the sequence and potentiometric levels tend to increase with depth (Walker and Mollica, 1990). Recharge occurs where these formations outcrop in the north of the basin and in the south of the basin along the Yarragon Fault, and also to the Thorpdale Volcanics that outcrop in the west of the basin. Recharge is from stream leakage and rainfall infiltration, including leakage from the Latrobe River in the north of the Gippsland Basin (Brumley and Holdgate, 1983). Groundwater flow is from these recharge areas towards the centre and towards the east of the basin where groundwater discharges via upwards leakage through the confining beds in the east of the basin, up into the watertable aquifer and the Latrobe River (Nicol, 2010). This upward leakage occurs as a result of elevated basement topography on the Haunted Hills Block, in combination with the lateral facies change from sandy to clayey sediments around the Moe Monocline, which restricts groundwater flow eastwards out of the basin and into the Latrobe Valley (Brumley and Holdgate, 1983; Nicol, 2010).
Aquifer depressurisation for coal mining and oil and gas development in the Gippsland Basin is not considered to have affected groundwater levels in the Moe Swamp sub-basin. This is due to the restricted hydraulic connectivity between the Moe Swamp sub-basin and the Latrobe Valley Depression resulting from the structuring in the east of the Moe sub-basin (Nicol, 2010).
Brumley and Holdgate (1983) described the Moe Swamp sub-basin as being in a steady state and noted that under higher rates of groundwater extraction, the structural elements of the basin would play a stronger role in groundwater flow.
Figure 28 shows a representative hydrograph of groundwater levels in the Moe Swamp sub-basin aquifers (bore 107970). Apparently higher abstraction may be evident from 1997 onwards with hydrographs indicating a transition to slightly lower groundwater levels from this time onwards.
1.1.4.3.4 Groundwater connectivity
As previously discussed, there is potential for inter-aquifer connectivity and leakage in the Gippsland Basin (Hofmann and Cartwright, 2013), however there remain significant knowledge gaps on the location and volumes of inter-aquifer leakage. A map of the distribution of aquitards was produced to indicate those areas of the groundwater system with the most potential for inter-aquifer connectivity (Figure 31).
Data: Victorian Government (Dataset 3), Victorian Government (Dataset 5), Bioregional Assessment Programme (Dataset 6), Bioregional Assessment Programme (Dataset 7)
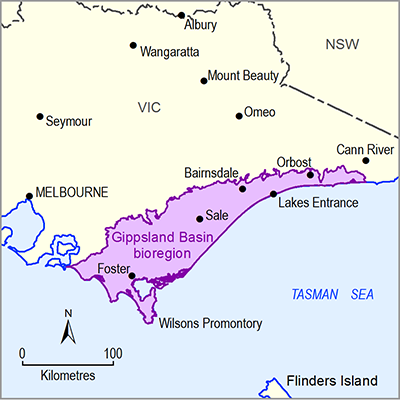