3.4.3.1 Description
Four landscape classes were defined in the ‘Riverine’ landscape group for the Hunter subregion, based on an unpublished flow regime classification approach from NSW Department of Primary Industries Water (companion product 2.3 for the Hunter subregion (Dawes et al., 2018)):
- permanent or perennial
- lowly to moderately intermittent
- moderately to highly intermittent
- highly intermittent or ephemeral.
Of the 3136 km of river in the zone of potential hydrological change, 634 km were determined to be permanent or perennial streams; 518 km lowly to moderately intermittent streams or moderately to highly intermittent streams; and 1985 km highly intermittent or ephemeral streams. During the development of qualitative and quantitative models via expert elicitation (see companion product 2.7 for the Hunter subregion (Hosack et al., 2018a)), the ‘Lowly to moderately intermittent’ landscape class and ‘Moderately to highly intermittent’ landscape class were collapsed into a single ‘Lowly to highly intermittent’ landscape class, which represents rivers that are variably connected to groundwater, irrespective of the frequency and duration of connection.
The ‘Permanent or perennial’ landscape class broadly corresponds to the ‘stable baseflow’ classes from Kennard et al. (2010; Classes 1, 2 and 3), while the ‘Lowly to moderately intermittent’ landscape class and ‘Moderately to highly intermittent’ landscape class (dealt with together as ‘Lowly to highly intermittent’ streams; see Table 4 in companion product 2.7 for the Hunter subregion (Hosack et al., 2018a)) correspond broadly to the ‘unpredictable baseflow’ and ‘intermittent’ classes from Kennard et al. (2010; Classes 4 and 5–8). Permanent or perennial streams have flow at least 80% of the year, and an appreciable contribution of groundwater to baseflows. Kennard et al. (2008) report baseflow indices in the range of 0.15 to 0.40 for perennial streams. Lowly to highly intermittent streams are characterised by streams that cease flowing more often than perennial streams and have a smaller baseflow contribution (0.07 to 0.25) due to an intermittent connection with groundwater (Kennard et al., 2008). Highly intermittent streams are characterised by an infrequent connection to groundwater and large numbers of zero-flow days.
River basins in the Hunter subregion include the Hunter, Macquarie-Tuggerah lakes, Upper Namoi and Lower Karuah. Only the Hunter river basin and Macquarie-Tuggerah lakes basin intersect the zone of potential hydrological change. The Hunter River is the largest river in the subregion and is fed by a number of significant tributaries, including Pages River, Dart Brook, Goulburn River (Figure 31), Glennies Creek, Wollombi Brook, Glendon Brook, Paterson River and Williams River. The total Hunter river basin area is 21,437 km2, of which 14,886 km2 is in the subregion. The Hunter River descends 1397 m over its 468 km course from its upper reaches in the Barrington Tops (outside the subregion), through the Hunter Valley, and out to sea.
Figure 31 The Goulburn River near Coggan
Source: Martin Krogh (2017). This figure is covered by a Creative Commons Attribution licence.
The Macquarie-Tuggerah lakes basin includes three main river basins: Dora Creek, Wyong River and Ourimbah Creek. The Macquarie-Tuggerah lakes basin covers an area of 1836 km2 and is bordered by the Hunter river basin in the north. Dora Creek runs south-east for 25 km to meet Lake Macquarie at the township of Dora Creek. The major tributaries of Dora Creek include Moran, Tobins, Jigadee, Blarney and Deep creeks. Wyong River runs south-east for 48 km to meet Tuggerah Lake at Tacoma. The Wyong River’s main tributaries include Jilliby Jilliby and Cedar Brush creeks. Ourimbah Creek runs south-east for 31 km to meet Tuggerah Lake at Chittaway. Ourimbah Creek’s major tributaries include Elliots, Bumbles, Toobys, and Bangalow creeks, which drain the southernmost corner of the subregion.
Ecologically important components of the hydrograph can be broadly summarised (Dollar, 2004; Robson et al., 2009) as cease-to-flow periods, periods of low flow, freshes, and periods of high flow (including overbench and overbank flows) as illustrated in Figure 32. Longitudinal, lateral and vertical connectivity is enhanced with increasing flow. Increasing flow increases connectivity between habitats and enables greater movement of aquatic biota, and water-borne nutrients and fine and coarse particulate organic matter. Flow regimes determine natural patterns of connectivity, which are essential to the persistence of many riverine populations and species (Bunn and Arthington, 2002). High flows are especially important for lateral connectivity and channel maintenance. Low flows are critical to maintaining vertical and longitudinal connectivity, and water quality of inundate habitat including pools. Freshes can trigger fish spawning, maintain water quality in inundate habitats, and cleanse and scour the riverbed. A lack of vertical connection to groundwater can result in cease-to-flow periods during periods of little or no rainfall. Cease-to-flow events dry out shallow habitats and can create chains of pools, isolated pools or completely dry riverbeds, depending on riverbed morphology (Robson et al., 2009). The limited lateral, vertical and longitudinal connectivity associated with the zero-flow condition is illustrated in Figure 33, where an isolated pool persists between dry riffle beds and the groundwater level is below the channel bed.
During periods of low flow (Figure 34), lateral connectivity is likely to be limited; however, low flows are important for maintaining vertical connectivity to the hyporheic zones of the streambeds (Ward, 1989; Kondolf et al., 2006), and for maintaining longitudinal connectivity within the landscape by linking instream habitats and allowing dispersal of instream biota (Dollar, 2004; Robson et al., 2009; Marsh et al., 2012; Boulton et al., 2014). Interactions between hydrological, ecological, and biogeochemical processes in the hyporheic zone influence key stream ecosystem processes in the surface stream, such as primary productivity and nutrient cycling (Boulton et al., 2010). The hyporheic sediments harbor microbes and invertebrates and are used by some fish for spawning.
Low flows provide seasonal habitat for many species and can maintain refugia for other species during droughts (Dollar, 2004). In regions with seasonal rainfall, low flows are maintained by baseflow, which is generally considered to be groundwater contribution to the hydrograph, hence the importance of the vertical connection of the riverbed to groundwater. A lack of vertical connection to groundwater can result in cease-to-flow periods during periods of little or no rainfall. Cease-to-flow events dry out shallow habitats and can create chains of pools, isolated pools or completely dry riverbeds, depending on riverbed morphology (Figure 33; Robson et al., 2009). In a synthesis of case studies Marsh et al. (2012) concluded that increasing durations of low flow are correlated with declining water quality (increased temperature and salinity and reduced dissolved oxygen), and that this a primary driver of ecological responses, especially in pools.
Riffle habitats are not only affected by changes in water quality but also reduced habitat area, as riffles dry out and contract. For example, Chessman et al. (2012) reported that aquatic macroinvertebrate assemblages that had been exposed to severe flow reduction or cessation during the period prior to sampling would be dominated by taxa tolerant of low oxygen concentrations, low water velocities and high temperatures, whereas assemblages not exposed to very low flows would be dominated by taxa that favour cool, aerated, fast-flowing conditions. Riffle habitats that are characterised by faster flowing, well-oxygenated water tend to be the first habitat type to be impacted by reduced river discharge. Marsh et al. (2012) also concluded that communities in streams that are usually perennial but cease to flow for short periods (weeks) will mostly recover the following season, but that the community composition will decline if cease to flow recurs over consecutive years.
Although lateral connectivity is limited under no- and low-flow conditions, riparian vegetation may directly access alluvial groundwater, in addition to perched watertables within the stream bank, or riverine water. The contribution of groundwater to evapotranspiration is likely important for maintaining function of the riparian vegetation (Dawson and Ehleringer, 1991) and may be higher during periods of low flow (Lamontagne et al., 2005).
Figure 34 Conceptual model of streams during periods of low flow when baseflows predominate
Freshes (Figure 35) are defined as flows greater than the median for that time of the year (Robson et al., 2009). They can last for several days and typically increase the flow variability within the stream as well as play an important role in the regulation of water quality through inputs of freshwater. Freshes can mobilise sediment, inundate larger areas of potential habitat, and connect in-channel habitats, thereby permitting migration of aquatic fauna (Robson et al., 2009). Freshes can increase vertical connectivity between the streambed and the hyporheic zone by scouring and cleansing the riverbed (Hancock and Boulton, 2005) and can also trigger spawning in some fish (King et al., 2009). Freshes increase lateral connectivity beyond that of low flows, and increase soil water availability in stream banks through increased bank recharge, helping to support the health and vigour of woody and herbaceous vegetation.
Figure 35 Conceptual model of streams during periods of freshes flow
The longitudinal connectivity is enhanced when compared to the low-flow conceptual model (Figure 34).
High flows (Figure 36 and Figure 37) inundate specific habitats and restore riverbed morphology (Robson et al., 2009). In the event of flooding they can also reconnect floodplains to the rivers and streams, fill wetlands, improve the health of floodplain trees and trigger waterbird breeding (Robson et al., 2009). High flows are often categorised ‘wet season baseflows’, ‘bank-full flows’ and ‘overbank flows’ (e.g. Robson et al. (2009)). For consistency with terminology used by experts during elicitation workshops (see Section 2.7.3.2 in companion product 2.7 for the Hunter subregion (Hosack et al., 2018a)), ‘overbench flow’ is used to represent both wet season baseflows and bank-full flows. A bench is bank-attached, narrow, relatively flat sediment deposit that develops between the riverbed and the floodplain.
Overbench flows partially or completely fill the channel for longer periods than freshes; typically weeks to months. Practically all habitat components within the river channel will be wetted, including boulders, logs and lateral benches (if present), and the entire length of the channel is connected with relatively deep water, allowing movement of biota along the river (Department of Sustainability and Environment, 2003). As for freshes, some native fish species rely on seasonal high flows during winter and spring as cues to start migration and prepare for spawning (Department of Sustainability and Environment, 2003), such as the diadromous and potamodromous species listed earlier.
Increased flow rates, such as during bank-full flows, scour banks and river substrate, and increase stream bank erosion. Bank erosion is accentuated under high discharge (bank-full condition), with the effectiveness of these erosional forces being a function of bank condition and the health of the riparian vegetation (Brierley and Fryirs, 2005), in addition to factors such as particle shape, density, packing, and biological activity such as algal growth (Boulton et al., 2014). Bank slumping or undercutting can create new habitats and contribute additional coarse woody debris to streams. Logs, sticks and root masses in the channel create depositional areas for sediment and for fine particulate organic matter. Localised increases in velocity profiles around snags scour out pools or undercut banks that provide habitat for large fish and other animals such as platypus (Boulton et al., 2014). Scouring of the benthic algal communities, often considered to be the main source of energy for higher trophic levels, can temporarily reduce stream primary production (Davie et al., 2012). Benthic algal communities often recover rapidly and grazing macroinvertebrates are able to feed preferentially on early succession benthic algal taxa, whereas late succession algae are less palatable or physically difficult to consume. High flow rates may also dislodge macrophytes and macroinvertebrates resulting in population drift downstream (Downes and Lancaster, 2010).
Overbank flows (Figure 37) inundate the surrounding floodplains, providing lateral connectivity, freshwater, nutrients and particulate matter to floodplain wetlands. These high-flow events also tend to enhance vertical connectivity providing a source of recharge for alluvial aquifers below the inundated floodplains (Doble et al., 2012) and recharge soil water reserves, which may promote seedling recruitment and promote health of the forested wetlands. However, Chalmers et al. (2009) also note that scouring of the floodplain can substantially increase seedling mortality. Connectivity to off-stream wetlands, via overbank flows, enables replenishment of freshwater in these systems, and migration of riparian floodplain biota to and from the main channel. In some agricultural environments, these processes may lead to high loads of nutrients being imported to the stream environment, which may have deleterious effects on instream habitats through algal blooms (Boulton et al., 2014).
Figure 36 Conceptual model of streams during periods of overbench flow
Dashed arrows represent high uncertainty in relation to the flux. The enhanced connectivity is relative to the freshes flow conceptual model (Figure 35).
Figure 37 Conceptual model of streams during periods of overbank flow
Dashed arrows represent high uncertainty in relation to the flux. The ‘high’ and ‘enhanced’ connectivity states are relative to the overbench flow (Figure 36).
3.4.3.2 Potential hydrological changes
3.4.3.2.1 ‘Permanent or perennial’ landscape class
The key hydrological determinants of ecosystem function identified by experts in the qualitative modelling workshops (companion product 2.7 for the Hunter subregion (Hosack et al., 2018a)) have been interpreted as a set of hydrological response variables. For the ‘Permanent or perennial’ landscape class, these are:
- number of zero-flow days per year, averaged over a 30-year period (ZQD, subsequently referred to in this section as ‘zero-flow days’)
- mean maximum spell duration of zero flow days over a 30-year period (ZME).
For details of these variables, see Section 2.7.3 in companion product 2.7 for the Hunter subregion (Hosack et al., 2018a).
There are about 634 km of perennial stream in the zone of potential hydrological change (based on a modelled flow regime classification). The vast majority of the perennial streams are not predicted to experience a change in ZQD of more than 3 days (Figure 38). Along the Hunter Regulated River, the surface water model was constructed to maintain a minimum flow rate of 80 ML/day, broadly consistent with the environmental water requirements under the Water Sharing Plan for the Hunter Regulated River Water Source 2016. Thus, results show no change in zero-flow days along the Hunter River. No increase in ZQD of more than 3 days is modelled to occur along the perennial Goulburn River either.
The only perennial stream where the increase in zero-flow days is predicted to exceed 3 days per year is in the Wyong River. Results from the regional hydrological modelling indicate at least a 5% chance that the mean number of zero-flow days per year will increase by fewer than 3 days and at least a 5% chance that increases of greater than 200 days per year will occur, with median estimates indicating increases between 20 and 80 days per year. However, as discussed in Section 3.3.3, when local hydrogeological information is used to constrain the result set from the hydrological modelling, the modelled estimates of increases in zero-flow days are much reduced, with increases of more than 13 days very unlikely, and median results indicating no change in the number of zero-flow days per year due to additional coal resource development.
Dora Creek in the Macquarie-Tuggerah reporting area is also potentially impacted. Dora Creek was not included in the surface water modelling network, but is identified in Section 3.6 as likely to experience changes in flow regime similar to what is predicted for Wyong River based on the modelled drawdowns. The hydrogeological information used to constrain model results in the Wyong River catchment might not be appropriate in the Dora Creek catchment, and further assessment of risk in the Dora Creek catchment should be informed by data from that catchment.
Foy Brook and Bayswater Creek are also potentially impacted. Part of Foy Brook flows through an area where there is a chance of drawdown due to additional coal resource development exceeding 2 m (Figure 19 in Section 3.3). It could experience changes in zero-flow days of at least 3 days and requires further investigation. Bayswater Creek flows through areas where the drawdown is very likely (greater than 95% chance) to be less than 2 m due to additional coal resource development. No definitive conclusion can be made about the magnitude of potential changes in zero-flow days due to additional coal resource development.
There is no discernible difference between changes in mean annual number of zero-flow days and mean maximum spell duration in ‘Permanent or perennial’ streams in 2042 (Figure 38) and 2102 (Figure 39).
The extent of the coal resource developments in the coal resource development pathway (CRDP) is the union of the extents in the baseline and in the additional coal resource development (ACRD).
Data: Bioregional Assessment Programme (Dataset 4, Dataset 5)
The extent of the coal resource developments in the coal resource development pathway (CRDP) is the union of the extents in the baseline and in the additional coal resource development (ACRD).
Data: Bioregional Assessment Programme (Dataset 4, Dataset 5)
3.4.3.2.2 ‘Lowly to highly intermittent’ landscape classes
Experts in the qualitative modelling workshops (companion product 2.7 for the Hunter subregion (Hosack et al., 2018a)) identified the same hydrological determinants of ecosystem function for the ‘Lowly to highly intermittent’ landscape class as for the ‘Permanent or perennial’ landscape class.
There are 518 km of intermittent stream in the zone of potential hydrological change (based on a modelled flow regime classification). Figure 40 shows the intermittent streams in the zone of potential hydrological change, classified by the magnitude of modelled change in mean number of zero-flow days (averaged over 30 years) (ZQD). Given the limitations of the interpolation (see Section 3.2.3.2), changes cannot be quantified for much of the intermittent stream network. The surface water model nodes on the intermittent streams are shown to provide the point estimates of modelled changes.
The majority of stream length is either very unlikely to be impacted or changes cannot be quantified. The upper Goulburn River and Wollar Creek are very unlikely to experience increases in zero-flow days, but the magnitude of potential changes in other intermittent streams are not quantified. Changes in zero-flow days in intermittent streams near all the additional coal resource developments cannot be ruled out.
There is a 50% chance of changes in zero-flow days in Saddlers Creek exceeding 20 days, and exceeding 3 days in Loders Creek and in an unnamed creek in the Bayswater Creek catchment. There is a 5% chance that increases in zero-flow days in Saddlers and Loders Creek will exceed 100 days. The regional-scale modelling identifies a risk in these streams. Local hydrogeological and catchment information, including water quality data, stream condition, habitat diversity, recovery potential and other catchment stressors (such as from baseline mines and other land uses) are needed to inform the true nature of the risk from the additional coal resource development.
There are small differences in the mean number of zero-flow days in ‘Lowly to highly intermittent’ streams in 2042 (Figure 40) and 2102 (Figure 41). Model nodes in Saddlers Creek and a tributary of Bayswater Creek indicate smaller changes in 2102.
The extent of the coal resource developments in the coal resource development pathway (CRDP) is the union of the extents in the baseline and in the additional coal resource development (ACRD).
Data: Bioregional Assessment Programme (Dataset 4, Dataset 5)
The extent of the coal resource developments in the coal resource development pathway (CRDP) is the union of the extents in the baseline and in the additional coal resource development (ACRD).
Data: Bioregional Assessment Programme (Dataset 4, Dataset 5)
3.4.3.3 Potential ecosystem impacts
As described in Section 3.4.2, most of the ‘Highly intermittent or ephemeral’ landscape class within the ‘Riverine’ landscape group is very unlikely to be impacted because it is assumed to not be connected to regional groundwater. In this section the potential ecosystem impacts on ‘Permanent or perennial’ streams and ‘Lowly to highly intermittent’ streams are discussed.
3.4.3.3.1 ‘Permanent or perennial’ landscape class
The receptor impact models for ‘Permanent or perennial’ streams are constructed around relationships between:
- mean abundance of larvae of the Hydropsychidae (net-spinning caddisflies) in a 1 m2 sample of riffle habitat and changes in zero-flow days (averaged over 30 years) (ZQD) and the mean maximum spell duration of zero flow days (ZME)
- mean probability of presence of the riffle-breeding frog (Mixophyes balbus) in a 100 m transect and changes in zero-flow days (ZQD) and the mean maximum spell duration of zero-flow days (ZME).
Hydropsychid caddisflies live in riffles because they rely on flowing water to feed. They play an important trophic role in many stream ecosystems, especially as a key food item for many fish. Median estimates of density of larvae of the Hydropsychidae under the baseline and coal resource development pathway (CRDP) ranged from 0 to 50 m-2 in a sample of riffle habitat (Figure 42). There is considerable uncertainty surrounding absolute values of density of larvae of the Hydropsychidae; there was a 90% chance that density could be in the range of 0 to more than 10,000 m-2 in both periods.
The median estimates of the difference in density of larvae of the Hydropsychidae due to additional coal resource development in the 30-year periods preceding 2042 and 2102 indicate no change from density under the baseline (Figure 42).
Based on the modelled 5th and 95th percentiles, potential increases or decreases in density of larvae of the Hydropsychidae are possible in some streams due to additional coal resource development. Potentially large changes in mean annual number of zero-flow days due to additional coal resource development in perennial streams are modelled to occur in Wyong River (Figure 38 and Figure 39) and, while not represented in the surface water modelling, potentially also Dora Creek where there is also a risk of extensive groundwater drawdown (Figure 19). The 5th percentile difference in Figure 42 reflects the risk to larvae of the Hydropsychidae from the modelled hydrological changes in Wyong River. Results indicate a 90% chance the impact on Hydropsychidae larvae will range between increases of up to 166 and decreases of up to 4100 caddisfly larvae per square metre of riffle habitat. The large range in predicted response indicates a high degree of uncertainty because other site factors influence the response to this perturbation.
In other perennial streams of the subregion, the modelled hydrological changes are very unlikely to impact larvae of the Hydropsychidae.
Data: Bioregional Assessment Programme (Dataset 6)
The stuttering frog (Mixophyes balbus) is a riffle-breeding frog, which breeds in streams during summer after heavy rain. Its eggs are laid on rock shelves or shallow riffles in small, flowing streams. The tadpoles are free-swimming benthic grazers, foraging amongst stones and leaf litter in riffle and pool sections of the stream channel (Anstis, 2002). As the tadpoles grow they move to deep, permanent pools and take approximately 12 months to metamorphose. Median estimates of probability of presence of the riffle-breeding frog (Mixophyes balbus) under the baseline and CRDP futures range from less than 0.1 to nearly 0.5 in a 100 m transect of riffle habitat (Figure 43). There is a lot of uncertainty around absolute values of probability of presence of the riffle-breeding frog (range of 0 to nearly 0.7 between 5th and 95th percentiles) in both periods.
The median estimate of change in probability of presence of the riffle-breeding frog due to additional coal resource development in the 30-year periods preceding both 2042 and 2102 indicates no change compared to the baseline period (Figure 43), except potentially in one small section of modelled stream. As for the Hydropsychidae larvae, the 5th and 95th percentiles show potential for increases or decreases in the probability of presence of the riffle-breeding frog in some streams due to additional coal resource development. Again, these large changes relate to the potentially large changes in zero-flow days modelled to occur in the Wyong River. Modelled decreases in the probability of presence of riffle-breeding frogs of up to nearly 0.5 indicate potential losses of instream habitat. The much smaller chance of changes in zero-flow days in the Wyong River catchment when local-scale hydrogeological data are used to constrain the regional model results (see Section 2.6.2.8 of companion product 2.6.2 for the Hunter subregion, Herron et al., 2018), however, suggest that the likelihood of significant adverse impacts on instream habitat is low.
Data: Bioregional Assessment Programme (Dataset 6)
In summary, model results suggest that across the subregion generally, impacts on much of the instream habitat of the ‘Permanent or perennial’ streams from hydrological changes due to additional coal resource development are very unlikely. There is at least a 5% chance that some ‘Permanent or perennial’ streams in the zone of potential hydrological change will experience adverse ecological impacts. The larger hydrological changes modelled in the Wyong River imply a risk to Hydropsychidae larvae and the probability of presence of riffle-breeding frog populations, and by extension to other components of the ecosystem that have similar water dependencies or depend on the presence of these populations for their persistence. When local information for the Wyong River catchment is incorporated into the groundwater and surface water modelling, the modelled hydrological changes are much smaller and hence the risk to instream habitat could be low. Other local information, relating to the presence and quality of instream habitats, the range of pressures upon them and their recovery potential, is needed to better inform the assessment of risk and guide the appropriate management response.
There is very high uncertainty surrounding estimates of absolute values. Uncertainties reflect not just lack of knowledge, but also the generalisation that is necessary in regional-scale assessments to capture the range of possible changes, which are conditioned on local-scale factors. Based on the qualitative model (see Section 2.7.3.2 in companion product 2.7 for the Hunter subregion (Hosack et al., 2018a)) increases in zero-flow days are likely to result in declines in invertebrates and other subsurface fauna, fish, and riparian and subsurface habitat and carnivores.
3.4.3.3.2 ‘Lowly to highly intermittent’ landscape classes
The receptor impact models for ‘Lowly to highly intermittent’ streams are constructed around relationships between:
- mean richness of hyporheic invertebrate taxa in 6 L of water pumped from a depth of 40 cm below the streambed (riffle and gravel bars) to changes in zero-flow days (averaged over 30 years) (ZQD) and the mean maximum spell duration of zero-flow days (ZME)
- mean probability of presence of the riffle-breeding frog (Mixophyes balbus) in a 100 m transect to changes in zero-flow days (ZQD) and the mean maximum spell duration of zero-flow days (ZME).
Median estimates of richness of hyporheic invertebrate taxa (hereafter referred to as hyporheic taxa) under the baseline and CRDP futures range from 13 to 15 in 6 L of water pumped from a depth of 40 cm below the streambed (Figure 44). There is a lot of uncertainty around absolute values of richness of hyporheic taxa (90% chance that richness of hyporheic taxa could be in the range of less than 4 to more than 40) in both periods.
The median estimate of change in richness of hyporheic taxa due to additional coal resource development in the 30-year periods preceding both 2042 and 2102 indicates that some intermittent streams might experience a reduction in mean richness of up to two taxa compared to the baseline period (Figure 44). Experts were confident that increases in zero-flow days would not result in increases in hyporheic taxa richness. There is at least a 5% chance of reductions in hyporheic taxa of up to ten taxa in some intermittent streams due to additional coal resource development. It is very unlikely that there will be decreases in hyporheic taxa richness in the upper Goulburn River and Wollar Creek due to additional coal resource development. There is at least a 50% chance of decreases in hyporheic taxa in Saddlers Creek and at least a 5% chance of decreases in Loders Creek. The condition of these streams, pressures from surrounding land uses and potential to recover are important considerations in determining an appropriate management response.
Hydrological changes were not modelled for many intermittent streams in the assessment extent (Figure 40 and Figure 41). Some are potentially impacted as they are in the groundwater drawdown zone. Impacts on the ecosystems in these streams cannot be ruled out.
Median estimates of probability of presence of the riffle-breeding frog (Mixophyes balbus) under the baseline and CRDP futures range from less than 0.12 to nearly 0.75 in a 100 m transect of riffle habitat (Figure 45). There is great uncertainty surrounding absolute values of probability of presence of the riffle-breeding frog; there is a 90% chance that the probability of presence of the riffle-breeding frog is between 0 and 1.
The median estimates from modelled changes in probability of presence of the riffle-breeding frog in the 30-year periods preceding both 2042 and 2102 indicate no change relative to the baseline period (Figure 45) in the majority of intermittent streams in the zone. The 5th and 95th percentiles show potential for increases or decreases in the probability of presence of the riffle-breeding frog in some of the modelled intermittent streams due to additional coal resource development. Based on the hydrological modelling results, the probability of presence of riffle-breeding frogs in Saddlers and Loders creeks is likely to be diminished due to additional coal resource development. Decreases in the probability of presence of riffle-breeding frogs of up to 0.4 are possible, which could mean very low probability of presence of frogs based on the baseline probabilities, or potentially complete loss of habitat. Loders and Saddlers creeks have relatively high stream salinities (medians >5500 μS/cm, Section 3.3.4); their catchments have already been disturbed by baseline and historical mining and agricultural development, and their geomorphic condition has been assessed as poor (Figure 46; NSW Office of Water, Dataset 7). Thus the habitat value of these creeks is likely to already be compromised, and the risk of adverse impacts from additional coal resource development is probably low. Local information on the factors influencing the hydrology of these creeks and the current condition of instream habitats is needed to put the regional-scale assessment into context for informing the appropriate management response.
In summary, the results suggest that for most of the modelled ‘Lowly to highly intermittent’ streams in the Hunter subregion, impacts on the instream habitat due to additional coal resource development are very unlikely. There is at least a 5% chance that some modelled ‘Lowly to highly intermittent’ streams in the zone will experience adverse ecological impacts, and by extension some of the non-modelled streams that drain or flow close to additional coal resource developments could also be impacted. The potentially large hydrological changes predicted in Saddlers Creek and Loders Creek could have adverse impacts on instream ecosystems, however, local stream salinity (Figure 28 and Figure 29) and geomorphic condition (Figure 46) information suggest they are already degraded.
There is considerable uncertainty surrounding estimates of absolute values. Based on the qualitative model (see Section 2.7.3.3 in companion product 2.7 for the Hunter subregion (Hosack et al., 2018a)) increases in the number of zero-flow days are likely to result in declines in invertebrates and other subsurface fauna, fish, and riparian and subsurface habitat and carnivores.
Data: Bioregional Assessment Programme (Dataset 6)
Data: Bioregional Assessment Programme (Dataset 6)
Figure 46 Geomorphic condition of streams in the Hunter assessment extent
Data: Bioregional Assessment Programme (Dataset 3, Dataset 4), NSW Office of Water (Dataset 7)
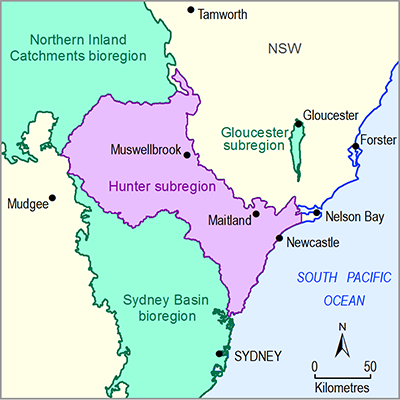
Product Finalisation date
- 3.1 Overview
- 3.2 Methods
- 3.3 Potential hydrological changes
- 3.4 Impacts on and risks to landscape classes
- 3.5 Impacts on and risks to water-dependent assets
- 3.6 Commentary for coal resource developments that are not modelled
- 3.7 Conclusion
- Citation
- Acknowledgements
- Contributors to the Technical Programme
- About this technical product