The centre of the Hunter subregion is located approximately 150 km north of Sydney in NSW, while the distance from the southern extent of the subregion to the Sydney GPO is only 36 km. It contains the agriculturally productive and key coal producing Hunter Valley and the Central Coast area, including Lake Macquarie, Tuggerah Lake and Brisbane Water. The subregion contains the major coastal cities of Newcastle in the north and Gosford in the south. Inland, towns such as Ulan border the western extent of the subregion and Murrurundi is located in the north. There are two features of high international conservation value including: (i) part of the Greater Blue Mountains World Heritage Area and (ii) the Hunter Estuary, a nationally and an internationally significant wetland listed under the Ramsar Convention on Wetlands (McCauley, 2006, Section 3.3 Land Use; NSW National Parks and Wildlife Service, 2003). The subregion is proximal to another two areas that also have significant international and local conservation value: (i) the Barrington Tops World Heritage Area (located directly north of the subregion) and (ii) the Myall Lakes National Park (located north-east of the subregion). In addition to these nationally and internationally recognised areas, the Hunter Valley is of great ecological significance as: (i) it represents the major break in the Great Dividing Range providing a link between coastal and inland NSW and (ii) it contains an area of overlap between tropical and temperate zones known as the MacPherson–Macleay Overlap (Burbidge, 1960) in which the limits of many taxa are found. For more details see Section 1.1.7 below.
The Hunter subregion is primarily defined by geological features (the Sydney Basin and the Hunter-Mooki fault) and ridge lines associated with the Hunter, Liverpool and Great Dividing ranges defining surface water catchments; see Section 1.1.1 for full details. It is approximately 230 km north–south and 210 km east–west. The 1:250,000 topographic dataset (Geoscience Australia, 2006) shows that several mountain ranges ring the subregion, including the Hunter, Liverpool and Great Dividing ranges. Land-surface elevation ranges from sea level to 1241 m (Australian Height Datum (AHD), Figure 8); it exceeds 1550 m (AHD) in the nearby Barrington Tops (located outside the subregion). The highest point in the subregion is East Bluff (1241 m), located in the Liverpool Range. Figure 9 shows that in the subregion the land surface slopes (calculated using ~90 m resolution grid cell – that is the 3 second Shuttle Radar Topography Mission (STRM; Farr et al., 2007) data) are flat to moderate in the undulating northern part of the subregion (along the Hunter River and its tributaries), with relatively rugged terrain found in the southern part of the subregion primarily associated with the heavily dissected rock outcrops of the Triassic Hawkesbury Sandstone. The maximum slope in the subregion is : 69 degrees, with some steep slopes being encountered in surrounding mountain areas. There are distinct break-of-slopes where the mountains and valley meet (Figure 9).
Figure 10 shows that from a surface water perspective the Hunter subregion is primarily composed of the Hunter river basin (87.5% of the subregion). River basins of the Macquarie and Tuggerah lakes comprise 10.7% of the subregion and the remaining 2% of the subregion comprises the Lower Karuah basin (1.3%) and the Upper Namoi basin (0.5%). Within the river basins of the Macquarie and Tuggerah lakes there are several natural coastal lakes including (i) Lake Macquarie, (ii) Tuggerah Lake, (iii) Brisbane Water, (iv) Budgewoi Lake and (v) Lake Munmorah that are important water bodies for recreation and habitat.
Figure 8 Surface elevation and mountain ranges of the Hunter subregion
Data: Geoscience Australia (Dataset 1), Geoscience Australia (Dataset 2), Geoscience Australia (Dataset 3)
Figure 9 Land surface slopes of the Hunter subregion
Data: CSIRO (Dataset 4)
Figure 10 Major river basins and selected surface water bodies of the Hunter subregion
The hydrogeological systems in the Hunter subregion are associated with Permian-Triassic rock aquifers, alluvial aquifers along major rivers and creeks and aeolian sands aquifer in the coastal zone of the subregion. The Hunter Valley represents a regional groundwater discharge zone and a dividing streamline for groundwater flow. Similarly to surface water, the main regional groundwater fluxes (south from Hunter-Mooki thrust fault, which forms the northern boundary of the geological Sydney Basin, and the Hunter subregion; see the previous section) largely follow the subregion topography from the upland towards the river channels with overall discharge towards the Tasman Sea. Interactions between surface water and groundwater in alluvial aquifers are important processes which determine quantity and quality of groundwater resources in the subregion. There are a series of connected alluvial deposits along the Hunter River and its tributaries, which form an important water resource. In water sharing plans the local alluvial aquifer is considered a separate water source for agriculture (DPI, 2005; 2013) with the Kingdom Ponds and Dart Brook alluvial aquifers, located in the north-west of the region, being the major groundwater resources. The Tomago Sandbeds are an important water resource for the Newcastle region (Woolley et al., 1995) being one of three main water supplies for this region. This aquifer provides a buffer against the effects of drought and could supply up to 30% of the water supply to areas of the lower Hunter River and Lake Macquarie. Other groundwater resources are largely dependent on the bedrock’s secondary permeability associated with fractures (Kellett et al., 1989). Water quality (salinity) limits use of groundwater found in Permian formations. Within the deeper strata of the Hunter subregion there is limited potential for groundwater interactions with groundwater systems to the south (the southern parts of the geological Sydney Basin that comprise the Sydney Basin Bioregional Assessment) and/or to the west (the geological Gunnedah Basin), but the volumetric fluxes between these basins are likely to be minimal. For more details about the hydrogeological systems see Section 1.1.4 .
Figure 11 shows the locations of wetlands and groundwater-dependent ecosystems in the Hunter subregion (Serov et al., 2012). The Hunter Estuary wetland is a nationally and internationally significant wetland listed under the Ramsar Convention on Wetlands. This wetland is a key site for migratory and resident shorebirds providing roosting and feeding resources to a large seasonal population of shorebirds and as a waylay site for transient migrants. Over 250 species of birds have been recorded within this Ramsar site, including 45 species listed under international migratory conservation agreements. In addition, the Hunter Estuary wetland provides habitat for three species nationally recognised as threatened: the green and golden bell frog, the red goshawk and the Australasian bittern; it also provides habitat for waterbirds such as ducks and herons during periods of inland drought. There are some important springs located in the Hunter subregion (Figure 11) which are key water sources for local ecosystems. Many of the rivers in the subregion have riparian vegetation along the banks, which provide important habitat for a variety of species. For more details about the ecology of the Hunter subregion see Section 1.1.7 .
The Hunter subregion is comprised of seven physiographic classes of the Australian Soil Resource Information System (ASRIS) (Figure 12). The main ASRIS classes are (i) Hunter Valley (covering 4946 km2 or 29.0% of the subregion), (ii) Hawkesbury-Shoalhaven Plateaus (covering 3980 km2 or 23.4% of the subregion), (iii) Merriwa Plateau (covering 3973 km2 or 23.2% of the subregion) and (iv) Goulburn Corridor (covering 3485 km2 or 20.4% of the subregion). The descriptions of all classes present in the subregion are provided in Table 6.
Figure 11 Wetlands and groundwater-dependent ecosystems of the Hunter subregion
Data: Serov et al. (2012), NSW Office of Water (Dataset 5)
Table 6 Description of the Australian Soil Resource Information System physiographic classes in the Hunter subregion, shown in Figure 12
Data: ASRIS (Dataset 6)
There are seven main soils found in the Hunter subregion – Tenosols (30.0%), Kurosols (27.3%), Ferrosols (13.1%), Sodosols (8.4%), Vertosols (8.3%), Rudosols (5.9%) and Dermosols (4.0 %) – together covering 97% of the subregion (Figure 13). Their characteristics are briefly introduced below, ordered by descending area.
Tenosols in the vicinity of the Hunter subregion are alluvial soils flanking rivers, so quite recently deposited. This means that they are young, weakly developed soils that have poorly developed (tenic) B horizons (Isbell, 2002). Given the location along rivers, these soils are likely to be dominated by clayey or silty textures, likely with pockets of sand or gravel present, and are probably deep.
Kurosols are located in mid- and lower slope positions. They have a clear, sharp, or abrupt textural boundary between coarser textured A horizons (e.g. sands or loams) and finer textured (i.e. clayey) B horizons (Isbell, 2002). The other distinguishing feature of these soils is that the upper 0.2 m of the B horizon is strongly acid (pH <5.5).
Ferrosols are located on upland landscape areas, and on crests, ridges or hill flanks. They are typically deeply red in colour reflecting a high concentration of free iron, and lack a strong contrast in texture between the topsoil and subsoil. Their structure is generally very good and if sufficiently deep, they are ideal for agriculture with appropriate erosion management.
Sodosols are generally located in lower hillsides or in perched upper slope locations. They are generally associated with salinity (e.g. at seeps or where drainage is poor), and salts can be of local origin (connate) or windblown. These soils have a strong contrast in textures between the topsoils and subsoil, with very clayey, poorly structured clay subsoil, and can be a challenge to manage for agriculture due to structural issues (caused by excessive sodium ions) and salinity.
Vertosols are generally uniform structured clay profiles which exhibit vertic properties (i.e. a subsoil with a field texture of 35% or more clay that experiences significant shrinking and swelling, resulting from drying and wetting), including surface cracks (often very deep and wide), self-mulching surface horizons and gilgai surface features (i.e. the land surface is irregular with alternating mounds and depressions due to clay horizons shrinking and swelling with alternate drying and wetting cycles). These soils are often found in alluvial landscapes where depositional energy is low or in situ on weathered, clay rich, flat lying, sedimentary rocks. The vertic properties arise from the shrink swell nature of the 2:1 layer clay minerals present and their response to wetting and drying.
Rudosols are generally associated with upper slopes, ridges and crests. These soils are poorly developed and typically young, so have had little time to develop structure. They may be deep or shallow, and either clayey, or loamy or sandy throughout the profile. Rudosols may also be stony.
Dermosols are likely to be dominated by clay that is near-uniform to slowly changing in texture in the profile (Isbell, 2002). These are well-structured soils and generally more clayey in the floodplains, where the deepest soils in the subregion are likely to be found.
Figure 13 Australian Soil Classification (ASC) classes in the Hunter subregion
Data: CSIRO (Dataset 7)
Figure 14 shows that prior to European settlement and its associated land clearing, the dominant overstorey vegetation in the Hunter subregion was: (i) canopy coverage in the range of 10 to 70%, (ii) eucalypt dominated and (iii) primarily of the tree or shrub growth-form (Carnahan (1976); Australian Survey and Land Information Group (AUSLIG) (1990)). Cool temperate rainforest dominated by species of Nothofagus were, and are still, present in the vicinity of the subregion (around the Barrington Tops; Figure 14).
Since European settlement there has been some vegetation clearing and the current major vegetation types are shown in Figure 15. Approximately one-half of the subregion contains remnant stands of native forests and woodlands, much of which are located in relatively rugged terrain associated with the southern part of the Hunter subregion, primarily associated with the heavily dissected Triassic capping Hawkesbury Sandstone. The Hunter subregion contains a number of Interim Biogeographic Regionalisation for Australia (IBRA) bioregions and subregions (SEWPaC, 2012b). The main IBRA bioregions within the Hunter subregion are the Sydney Basin bioregion (77% of the Hunter subregion; see the previous section) and the Brigalow Belt South bioregion (20% of the Hunter subregion; see the previous section). The remaining 3% of the Hunter subregion is covered by the NSW North Coast and Nandewar IBRA bioregions.
The Sydney Basin IBRA bioregion is one of the most species-diverse in Australia owing to great variation in geology, topography and climates (NSW National Parks and Wildlife Service, 2003). Over 38% of this IBRA bioregion is in conservation-oriented tenures, primarily national parks and conservation reserves. Over 15% is composed of six wilderness areas. The Greater Blue Mountains World Heritage Area, which occupies over 28% of the Sydney Basin IBRA bioregion, also contains the highly significant Wollemi National Park, protecting threatened species, many of which are locally endemic. Discovered in September 1994, the Wollemi pine (Wollemia nobilis) occurs only in a remote canyon in the park. Plant communities within the Sydney Basin IBRA bioregion include: (i) estuarine mangroves, salt marshes and reed swamps, (ii) coastal dune communities of eucalypt, banksia, tea-tree, she-oak, wattle or applebark, (iii) rainforest communities in gullies and canyons, (iv) coastal forest of Sydney bluegum, forest and open woodlands dominated by eucalypts and applebarks and (vi) river oaks and river red gums along streams. The Sydney Basin IBRA bioregion also contains nine significant wetlands. Further details of the vegetation of the Sydney Basin IBRA bioregion at the IBRA subregion level are provided in Section 1.1.7 .
The part of the Brigalow Belt South IBRA bioregion within the Hunter subregion is almost entirely within the Liverpool Plain subregion of the Brigalow Belt South IBRA bioregion (NSW National Parks and Wildlife Service, 2003). Within the Liverpool Plains IBRA subregion, the threatened plant community of diverse grasslands with box trees and wilga occurs on black earths, whereas texture contrast hillslope soils are characterised by white box and white cypress pine with rough-barked apple, hill red gum, and occasional belah and mulga.
Figure 14 Vegetation prior to European settlement in the Hunter subregion
Data: Department of Environment (Datatset 8), AUSLIG (1990)
Figure 15 Current major vegetation types in the Hunter subregion
Data are not available for the entire map frame area, these are represented by white
Data: NSW Office of Environment and Heritage (Dataset 9)
Land cover is best observed from satellite imagery, which provides the opportunity to understand dynamics, calculate long-term averages and to determine the relative contributions from persistent and recurrent vegetation types (Donohue et al., 2009). Figure 16 shows that the total vegetation cover, derived from the MODIS satellite instrument for 2000 to 2012, for most of the Hunter subregion varies from 50 to 100%, with some areas having low total vegetation cover being associated with open-cut mining and urban areas. Much of the vegetation cover is persistent, in that it is ‘green’ for most of the year, and is associated with the remnant stands of native forests previously discussed. Figure 16 shows that only a relatively small proportion of the vegetation has a strong annual signal that defines recurrent (e.g. cropping) vegetation, where land cover varies from bare soil (i.e. zero % vegetation cover) to exceeding approximately 50% vegetation cover over a three to four month period (Figure 16). Within the Hunter subregion this pulse of recurrent vegetation is associated with cropping systems located in the western part of the subregion. The mean monthly dynamics of the recurrent component, illustrating strong ‘spring’ growth from July to October, are provided in Figure 17 .
Vegetation height can be derived from satellite measurements/data, specifically lidar (light detection and ranging) (Simard et al., 2011) and, using this data source, Figure 18 shows that the persistent vegetation encountered over much of the Hunter subregion is tall (i.e. ~20 to 40 m high in the 1 km resolution grids calculated using data captured in 2005). While almost 40% of the land use in the subregion is grazing (Figure 20), there are isolated remnant mature trees (providing shade for livestock) in paddocks. These trees are causing some cells in pastures to have higher than expected mean cell heights.
Figure 16 Vegetation cover in the Hunter subregion
Long-term mean values from 2000 to 2012 derived from the MODIS satellite sensor (Paget and King, 2008) with a 250 m grid cell resolution are shown. Total cover is temporally decomposed to provide persistent and recurrent estimates using the method of Donohue et al. (2009).
Data: CSIRO (Dataset 10)
Figure 17 Monthly recurrent land cover in the Hunter subregion
Long-term monthly mean values from 2000 to 2012 derived from the MODIS satellite sensor (Paget and King, 2008) with a 250 m grid cell resolution are shown. Total cover is temporally decomposed to provide persistent and recurrent estimates using the method of Donohue et al. (2009).
Data: CSIRO (Dataset 10)
Figure 18 Vegetation height of the Hunter subregion
Data: CSIRO (Dataset 11)
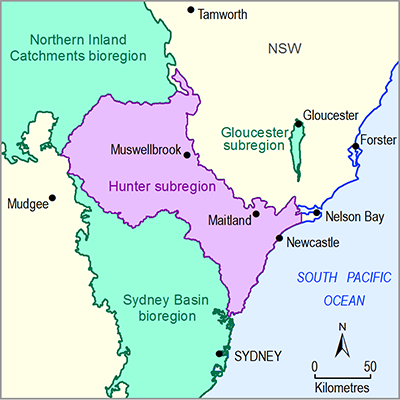