Companion product 2.3 for the Gloucester subregion describes seven landscape classes within the ‘Riverine’ landscape group in the assessment extent (AE) (Dawes et al., 2018):
- Intermittent – gravel/cobble streams
- Intermittent – high gradient bedrock confined streams
- Intermittent – lowland fine streams
- Perennial – gravel/cobble streams
- Perennial – high gradient bedrock confined streams
- Perennial – lowland fine streams
- Perennial – transitional fine streams.
No moderately intermittent or ephemeral streams were identified as landscape classes in the AE for the Gloucester subregion. The perennial river landscape classes broadly correspond to the ‘stable baseflow’ classes from Kennard et al. (2010; Classes 1, 2 and 3), while the intermittent river landscape classes correspond broadly to the ‘unstable baseflow’ and ‘rarely intermittent’ classes from Kennard et al. (2010; Classes 4 and 5). Perennial streams have flow at least 80% of the year, and an appreciable contribution of groundwater to baseflows. Kennard et al. (2008) reported a baseflow index of 0.15 to 0.4 for perennial streams. Intermittent streams cease flowing more often than perennial streams and have a lesser (0.12 to 0.25) baseflow contribution (Kennard et al., 2008).
The two closely related landscape classes, ‘Perennial – gravel/cobble streams’ and ‘Intermittent – gravel/cobble streams’, overwhelmingly dominate potentially impacted stream reaches in the ‘Riverine’ landscape group (see Section 2.7.2). The ‘Perennial – gravel/cobble streams’ landscape class represents 52% of the stream network within the non-estuarine region of the AE (refer to companion product 2.3 for the Gloucester subregion (Dawes et al., 2018)). In the northern half of the AE, the landscape class is mainly restricted to the Gloucester River; in the southern half of the AE it also occurs along the Karuah and Mammy Johnsons rivers. The ‘Intermittent – gravel/cobble streams’ landscape class occupies 20% of the stream network within the non-estuarine region of the AE, with the majority occurring in the northern half of the AE (companion product 2.3 for the Gloucester subregion (Dawes et al., 2018)). This landscape class is dominated by the Avon River (which itself is a major tributary of the Gloucester River) and its tributaries. The Avon river basin area occupies approximately 73% of the northern-flowing part of the AE, and descends 412 m over its 42 km course (companion product 1.1 for the Gloucester subregion (McVicar et al., 2014)). Hence, receptor impact modelling for the ‘Riverine’ landscape group focused on the ‘Perennial – gravel/cobble streams’ and ‘Intermittent – gravel/cobble streams’ landscape classes, and a conceptual model for the two classes is presented in this section.
Pools and riffles are most common in streams with mixed bed materials ranging from 2 to 256 mm in size (Knighton, 1984); hence, they are a feature of the ‘Perennial – gravel/cobble streams’ and ‘Intermittent – gravel/cobble streams’ landscape classes. The mixed substrate favours formation of alternating pool and riffle sequences that increase the geomorphic and habitat complexity along the reach (Boulton et al., 2014). Riparian vegetation lining the banks of the riverine landscape classes provides important instream and terrestrial habitats and contributes to geomorphic condition by maintaining bank stability (Boulton et al., 2014). Within the Gloucester zone of potential hydrological change, this riparian vegetation is dominated by forested wetlands, typically characterised by a eucalypt-dominated overstorey and a grassy or shrubby understorey, although actual species composition is highly variable (Keith, 2004). The Karuah River from Stroud to Karuah is relatively well vegetated but much of the riparian zone is cleared along the Avon and Gloucester rivers (see Section 1.1.7.2 of companion product 1.1 for the Gloucester subregion (McVicar et al., 2014)) and much of the riparian zone outside of the Karuah National Park and reserves is in poor condition (Haine et al., 2012). Patches of rainforest, including the ‘Lowland Rainforest of Subtropical Australia’ threatened ecological community, are largely restricted to reaches along the Karuah River (see Section 2.3.3.1 of companion product 2.3 for the Gloucester subregion (Dawes et al., 2018)).
Hydrological regimes for the AE for the Gloucester subregion are discussed in more detail in companion product 1.1 (McVicar et al., 2014) and companion product 2.1-2.2 (Frery et al., 2018) and only a brief summary of these is presented here for context. There are no surface water connections between the northern and southern halves of the subregion, and no evidence of substantial groundwater connection between the two. Groundwater monitoring undertaken by Parsons Brinckerhoff (2012a, 2012b, 2013a) within the geological Gloucester Basin provides some evidence for a topographical shallow groundwater flow divide in the middle of the basin north of Wards River, approximately coincident with the surface water divide. This separates the Gloucester Basin into a northern sub-basin (where regional groundwater flow is predominantly from south to north) and a southern sub-basin (where regional groundwater flow is predominantly from north to south) (companion product 1.1 for the Gloucester subregion (McVicar et al., 2014)).
Stream and aquifer salinity indicate that on average, streams within the AE for the Gloucester subregion are gaining; thus, groundwater provides an important source of baseflow in this landscape group (companion product 1.1 for the Gloucester subregion (McVicar et al., 2014)). Groundwater recharge was estimated as zero to 17% (under steady-state conditions) and zero to 28% (under transient conditions) of rainfall, with high values associated with alluvial aquifers (Australasian Groundwater and Environmental, 2013; Heritage Computing, 2009, 2012; Parsons Brinckerhoff, 2013b). Discharge occurs as localised discharge to rivers and streams and as diffuse discharge, via evapotranspiration, from deep-rooted vegetation. Groundwater salinity increases with depth, although typically, groundwater associated with alluvial aquifers varies from fresh to brackish (electrical conductivity (EC) of 387 to 5810 µS/cm; companion product 1.1 for the Gloucester subregion (McVicar et al., 2014)). Companion product 2.1-2.2 for the Gloucester subregion (Frery et al., 2018) reported that groundwater depths in the alluvium ranged from very near the surface (<0.1 m) to 13.4 m below ground level. Almost no data were available outside the alluvium; however, modelled depths to water (Summerell and Mitchell, 2011) suggest that groundwater is not deeper than 12 to 16 m in any of the lowland areas of the AE (Figure 8).
In the northern half of the AE, approximately 43 km of the middle and lower reaches of the Gloucester River occur within the subregion. In the north of the AE, average annual streamflow and baseflow index (calculated using digital filtering) are 550 GL/year and 0.58 for the gauge at Doon Ayre (208003), respectively (Figure 7). At the Gloucester stream gauge (208020), average annual streamflow and baseflow index are 84 GL/year and 0.56, respectively. There is high interannual variability in high-flow regimes related to rainfall inputs. Baseflows tend to be less variable due to the buffering effects of groundwater inputs (see the surface water hydrology section in companion product 1.1 (McVicar et al., 2014) and companion product 2.1-2.2 for the Gloucester subregion (Frery et al., 2018)). The Barrington River discharges approximately 435 GL/year at the Relf Rd streamflow gauge (208031) located about 1 km upstream from its confluence with the Gloucester River. Stream cross-sections and flow duration curves at the stream gauges for the Gloucester River at Gloucester, Relf Rd and Doon Ayre are shown in Figure 7. At the Gloucester stream gauge, high overbank flows are rare, occurring with an average frequency of 1 in 27 years (overbank threshold ~10 GL/day). Cease-to-flow periods are also extremely rare, occurring at an average frequency of approximately once in 127 years. Streamflow peaks tend to occur in the late summer–autumn period and decline over winter to a minimum in spring (companion product 1.3 for the Gloucester subregion (McVicar et al., 2015)) (Table 6). Only one stream gauge occurs in the ‘Intermittent – gravel/cobble streams’ landscape class, on the Avon River at Waukivory (208028). Maximum monthly flows occur in February and minimum flows occur in January (companion product 1.1 (McVicar et al., 2014)). The overbank threshold for this stream gauge is 3621 GL, occurring at an average frequency of twice per year. Cease-to-flow periods are very frequent, occurring at an average frequency of 26.5 days per year. Mean annual flow at the stream gauge is 96 GL/year and the baseflow index is 0.26. The river cross-section and flow duration curve for Avon River at Waukivory are shown in Figure 7.
In the south of the AE, the ‘Perennial – gravel/cobble streams’ landscape class is largely confined to the Karuah and Mammy Johnsons rivers. There are two stream gauges (209003 Karuah River at Booral; 209002 Mammy Johnson) located within the ‘Perennial – gravel/cobble streams’ landscape class in the southern half of the AE. Annual streamflow and the baseflow index for the Karuah at Booral are 270 GL/year and 0.50, respectively (companion product 1.1 (McVicar et al., 2014)). Mean annual streamflow and baseflow index for the stream gauge at Mammy Johnsons are 56 GL/year and 0.33, respectively. The overbank threshold for this stream gauge is 24 GL, occurring at an average frequency of once every 2.7 years. The cease-to-flow frequency is also higher than in the Gloucester River with a cease-to-flow frequency of once every 1.4 years. Seasonal patterns in the streamflow peaks and minima are similar to that described above, although the maxima and minima vary between stream gauges (Table 6).
The locations of these stream gauge stations are shown on Figure 27 in companion product 1.1 for the Gloucester subregion (McVicar et al., 2014).
Source: NSW Office of Water (2015)
Table 6 Maximum and minimum flow regimes for stream gauges in ‘Perennial – gravel/cobble streams’ and ‘Intermittent – gravel/cobble streams’ landscape classes in the Gloucester subregion
Figure 8 Depth to watertable in the assessment extent for the Gloucester subregion
Data: NSW Department of Primary Industries (Dataset 1)
2.7.3.1.1 Flora and fauna
Only 3% of the perennial stream length and 2% of the intermittent stream length within the zone of potential hydrological change were classed as being in good geomorphic condition (Figure 9; NSW Office of Water, Dataset 2). Chessman et al. (2006) observed that river reaches of the Bega River in good geomorphic condition were important for maintaining native biodiversity and were biologically very different from stream reaches in moderate or poor condition. Deterioration from moderate to poor geomorphic condition resulted in less biological change than the deterioration from good to moderate. Of the intermittent streams within the zone of potential hydrological change, 51% were in moderate condition and 46% were in poor condition. Of the perennial streams within the zone, 83% were in moderate condition and 15% were in poor condition. Of the intermittent river reaches within the zone, 59% had some vegetation cover, while 88% of the perennial river reaches had some vegetation cover (Figure 10).
Riparian vegetation (Figure 10) along both perennial and intermittent streams is dominated by Waterhousea floribunda/Tristaniopsis laurina riparian warm temperate rainforest and Casuarina cunninghamiana/Melia azedarach grassy riparian forest (Keith, 2004). Other vegetation associated with these streams includes overstorey trees such as Angophora costata, Corymbia maculata, Eucalyptus amplifolia, E. canaliculata, E. grandis, E. punctata, E. saligna, E. tereticornis and Ficus coronata. E. tereticornis is considered to be a primary feed tree for koalas in the central coast region (NSW Office of Environment and Heritage, 2016).
Rainforest patches may provide important habitat for rainforest birds such as the superb fruit-dove (Ptilinopus superbus), and include overstorey tree species such as Lophostemon suaveolens, Livistona australis and Melaleuca sp. Understorey shrubs include cheese tree (Glochidion ferdinandi), swamp paperback (Melaleuca ericifolia) and waterbush (Myoporum acuminatum). The grass layer includes a range of forbs, herbs, sedges and grasses (Keith, 2004).
These vegetation associations may form habitat for several threatened plant species included in the asset register for the Gloucester subregion (see companion product 1.3 for the Gloucester subregion (McVicar et al., 2015)), including the Charmhaven apple (Angophora inopina), white-flowered wax plant (Cynanchum elegans), leafless tongue orchid (Cryptostylis hunteriana), slaty redgum (Eucalyptus glaucina) and trailing woodruff (Asperula asthenes). In addition, riparian vegetation can form habitat for a range of vertebrate and invertebrate fauna. Examples of vertebrate fauna from the asset register listed under the Commonwealth’s Environment Protection and Biodiversity Conservation Act 1999 (EPBC Act) that might use riparian vegetation (e.g. forested wetlands), either for habitat or feeding, include the grey-headed flying-fox (Pteropus poliocephalus), red goshawk (Erythrotriorchis radiatus), regent honeyeater (Anthochaera phrygia), swift parrot (Lathamus discolor) and giant barred frog (Mixophyes iteratus) (CSIRO, Dataset 3). The ‘Riverine’ landscape group includes platypus habitat (an asset listed in the asset register for the Gloucester subregion). The streams and their associated riparian vegetation provide migration corridors for aquatic and terrestrial fauna and habitat for a range of threatened species, such as the spot-tailed quoll (Dasyurus maculatus subsp. maculatus).
It is important to note that there is large uncertainty around the mapping of individual plant-community types in the Gloucester subregion; a recent ground-truth study in the Upper Hunter (Hunter, 2015) found that only 7% of plant-community types were reliably mapped. Even at the level of vegetation formation, only dry sclerophyll forests and woodlands were mapped with greater than 50% accuracy. On this basis, the landscape classes were not defined at finer hierarchical levels such as vegetation class or plant-community type.
Figure 9 (a) Flow regime and (b) geomorphic condition of streams in the Gloucester subregion
Data: NSW Office of Water (Dataset 2)
ACRD = additional coal resource development, CSG = coal seam gas, GDE = groundwater-dependent ecosystem
Data: NSW Office of Water (Dataset 2)
The ‘Riverine’ landscape group supports a range of native and introduced fish species. Native species that migrate upstream and downstream may be important indicators of longitudinal connectivity (Koehn and Crook, 2013). Examples include the diadromous (migrates between fresh and estuarine waters) Australian bass (Macquaria novemaculeata) and striped gudgeon (Gobiomorphus australis), and the potamodromous (migrates wholly within fresh waters) Cox’s gudgeon (Gobiomorphus coxii). Changes to flow regimes may impact the life cycles of these fish by (NSW Department of Primary Industries, 2006):
- interrupting spawning or seasonal migrations
- restricting access to preferred habitat and available food resources
- reducing genetic flow between populations
- increasing susceptibility to predation and disease through accumulations below barriers
- fragmenting previously continuous communities
- disrupting downstream movement of adults and impeding larval drift through the creation of still water (lentic) environments.
Other native fish species reported from NSW DPI fisheries monitoring (NSW Department of Primary Industries, Dataset 4) of the Gloucester, Karuah, Wards and Mammy Johnsons rivers include Australian smelt (Retropinna semoni), bullrout (Notesthes robusta), common jollytail (Galaxias maculatus), dwarf flathead gudgeon (Philypnodon macrostomus), empire gudgeon (Hypseleotris compressa), firetail gudgeon (Hypseleotris galii), flathead gudgeon (Philypnodon grandiceps), eel-tailed catfish (Tandanus tandanus), freshwater herring (Potamalosa richmondia), freshwater mullet (Myxus petardi), long-finned eel (Anguilla reinhardtii), sea mullet (Mugil cephalus), short-finned eel (Anguilla australis), southern blue-eye (Pseudomugil signifer) and yellowfin bream (Acanthopagrus australis). Exotic fish species have also been observed (e.g. eastern gambusia and goldfish).
2.7.3.1.2 Ecologically important flows
Ecologically important components of the hydrograph can be broadly summarised (Dollar, 2000; Robson et al., 2009) as cease-to-flow periods, periods of low flow, freshes, and periods of high flow (including overbench and overbank flows) as illustrated in Figure 11. Longitudinal, lateral and vertical connectivity is enhanced with increasing flow. Increasing flow increases connectivity between aquatic habitats and enables greater movement of aquatic biota and water-borne nutrients, and fine and coarse particulate organic matter. Flow regimes determine natural patterns of connectivity, which are essential to the persistence of many riverine populations and species (Bunn and Arthington, 2002). High flows are especially important for lateral connectivity and channel maintenance. Low flows are critical to maintaining vertical and longitudinal connectivity, and water quality of inundated habitat including pools. Freshes can trigger fish spawning, maintain water quality in inundated habitats and cleanse and scour the riverbed.
A lack of vertical connection to groundwater can result in zero-flow periods (Figure 12) during periods of little or no rainfall. Cease-to-flow events dry out shallow habitats and can create chains of pools, isolated pools or completely dry riverbeds, depending on riverbed morphology (Robson et al., 2009). Chessman et al. (2012) reported that aquatic macroinvertebrate assemblages that had been exposed to severe flow reduction or zero flow during the period prior to sampling would be dominated by taxa tolerant of low oxygen concentrations, low water velocities and high temperatures, whereas assemblages not exposed to very low flows would be dominated by taxa that favour cool, aerated, fast-flowing conditions. Riffle habitats that are characterised by faster flowing, well oxygenated water tend to be the first habitat type to be impacted by reduced river discharge. Marsh et al. (2012) also concluded that communities in streams that are usually perennial but cease to flow for short periods (weeks) will mostly recover the following season but that the community composition will decline if cease-to-flow periods recur over consecutive years.
During periods of zero flow and low flow (Figure 12 and Figure 13), lateral connectivity is likely to be limited; however, low flows are important for maintaining vertical connectivity to the hyporheic zones of the streambeds (Ward, 1989; Kondolf et al., 2006), and for maintaining longitudinal connectivity within the landscape by linking instream habitats and allowing dispersal of instream biota (Dollar, 2000; Robson et al., 2009; Marsh et al., 2012; Boulton et al., 2014). Low flows (Figure 13) provide seasonal habitat for many species and can maintain refugia for other species during droughts (Dollar, 2000). In regions with seasonal rainfall, low flows are maintained by baseflow, which is generally considered to be a groundwater contribution to the hydrograph, hence the importance of the vertical connection of the riverbed to groundwater. In a synthesis of case studies, Marsh et al. (2012) concluded that increasing durations of low flow are correlated with declining water quality (increased temperature and salinity and reduced dissolved oxygen), and that this a primary driver of ecological responses of most aquatic biota, especially in pools. Riffle habitats are not only affected by changes in water quality but also by reduced habitat area, as riffles dry out and contract.
Although lateral connectivity is limited under zero-flow and low-flow conditions, riparian vegetation may directly access alluvial groundwater, in addition to accessing perched watertables within the stream bank and directly accessing riverine water. The contribution of groundwater to evapotranspiration is likely important for maintaining function of the riparian vegetation (Dawson and Ehleringer, 1991) and may be higher during periods of low flow (Lamontagne et al., 2005).
Figure 13 Conceptual model of streams during periods of low flow when baseflows predominate
Freshes (Figure 14) are defined as flows greater than the median for that time of the year (Robson et al., 2009). They can last for several days and typically increase the flow variability within the stream as well as playing an important role in the regulation of water quality through inputs of fresh water. Freshes can mobilise sediment, inundate larger areas of potential habitat, and connect in-channel habitats – thereby permitting migration of aquatic fauna (Robson et al., 2009). Freshes can increase vertical connectivity between the streambed and the hyporheic zone by scouring and cleansing the riverbed (Hancock and Boulton, 2005), and can trigger spawning in some fish (King et al., 2009).
Figure 14 Conceptual model of streams during freshes
The longitudinal connectivity is enhanced when compared to the low-flow conceptual model (Figure 13).
High flows (Figure 15 and Figure 16) inundate specific habitats and can alter riverbed morphology (Robson et al., 2009). In the event of flooding, they can also reconnect floodplains to the rivers and streams, fill wetlands, improve the health of floodplain trees and trigger waterbird breeding (Robson et al., 2009). High flows are often categorised as ‘wet season baseflows’, ‘bank-full flows’ and ‘overbank flows’ (e.g. Robson et al., 2009). For consistency with terminology used by experts during elicitation workshops (see Section 2.7.3.2), the term ‘overbench flow’ is used here to represent both wet season baseflows and bank-full flows. A bench is a bank-attached, narrow, planar sediment deposit that develops between the riverbed and the floodplain.
Overbench flows partially or completely fill the channel for longer periods than freshes – typically weeks to months. Practically all habitats within the river channel will be wetted including boulders, logs, and lateral benches (if present), and the entire length of the channel is connected with relatively deep water, allowing movement of biota along the river (Department of Sustainability and Environment, 2003). As for freshes, some native fish species rely on seasonal high flows during winter and spring as cues to start migration and prepare for spawning (Department of Sustainability and Environment, 2003), such as the diadromous and potamodromous species listed in Section 2.7.3.1.1.
Increased flow rates, such as during bank-full flows, scour banks and river substrate, and increase erosion of banks. Bank erosion is accentuated under high discharge (bank-full condition), with the effectiveness of these erosional forces being a function of bank condition and the health of the riparian vegetation (Brierley and Fryirs, 2005), in addition to factors such as particle shape, density, packing and biological activity such as algal growth (Boulton et al., 2014). Bank slumping or undercutting can create new habitats and contribute additional coarse woody debris to streams. Logs, sticks and root masses in the channel create depositional areas for sediment and for particulate organic matter. Localised increases in velocity profiles around snags scour out pools or undercut banks that provide habitat for large fish and other organisms such as platypus (Boulton et al., 2014). Scouring of the benthic algal communities, often considered to be an important source of energy for higher trophic levels, can temporarily reduce stream primary production (Davie et al., 2012); however, benthic algal communities typically recover rapidly and many grazing macroinvertebrates feed preferentially on early-succession benthic algal taxa, whereas late-succession algae are less palatable or physically difficult to consume. High flow rates may also dislodge macrophytes and macroinvertebrates, resulting in population drift downstream (Downes and Lancaster, 2010).
Overbank flows (Figure 16) inundate the surrounding floodplains, providing lateral connectivity, fresh water, nutrients and particulate matter to floodplain wetlands. In the Gloucester subregion, the surrounding floodplains are extensively cleared for agriculture (companion product 1.1 for the Gloucester subregion (McVicar et al., 2014)). These high-flow events also tend to enhance vertical connectivity, providing a source of recharge for alluvial aquifers below the inundated floodplains (Doble et al., 2012) and recharge soil water reserves, which may promote seedling recruitment and maintain the health of the forested wetlands. However, Chalmers et al. (2009) also note that scouring of benches and bars can substantially increase seedling mortality. Connectivity to offstream wetlands, via overbank flows, enables replenishment of fresh water in these systems, and migration of riparian floodplain biota to and from the main channel. In some agricultural environments, these processes may lead to high loads of nutrients being imported to the stream environment, which may have deleterious effects on instream habitats through algal blooms (Boulton et al., 2014).
Figure 15 Conceptual model of streams during periods of overbench flow
Dashed arrow represents high uncertainty in relation to the flux. The enhanced connectivity is when compared to the freshes flow conceptual model (Figure 14).
Figure 16 Conceptual model of streams during periods of overbank flow
The ‘high’ and ‘enhanced’ connectivity states are relative to the overbench flow conceptual model (Figure 15).
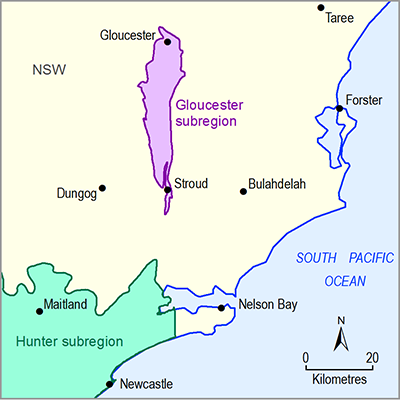