- Home
- Assessments
- Bioregional Assessment Program
- Methods
- Receptor impact modelling
- 1 Background and context
Receptor impact modelling is integral to undertaking the impact and risk analysis of a bioregional assessment (BA). The Methodology for bioregional assessments of the impacts of coal seam gas and coal mining development on water resources (the BA methodology; Barrett et al., 2013) states:
Modelling and analysis of the direct, indirect and cumulative impacts of coal seam gas (CSG) and coal mining development on anthropogenic and ecological receptors is the pivotal component of a BA.
The receptor impact model predicts how a receptor impact variable (a variable for which water-related impacts on assets are to be assessed) responds to direct, indirect and cumulative impacts of CSG and coal mining development. Although the BA methodology gives a high-level overview of the components required for a BA, it is not sufficiently detailed to clearly guide Assessment teams performing specific assessment tasks. This submethodology provides the explicit detail needed to develop the receptor impact models that are required to assess the potential impacts from CSG and large coal mining on receptor impact variables. This M08 report is a methodology report that provides the technical detail underlying the reporting in technical products 2.7 and 3-4. A quantitative, numerical and probabilistic modelling approach is described. The Assessment teams can use this method to undertake probabilistic assessment for a receptor impact variable as required by BA. The receptor impact modelling methodology was purposely developed to enable coherent assimilation of empirical data if permitted by available resources within the BA Programme. The methodology describes the generation of a probabilistic prior that can be updated with empirical data where available.
Receptor impact models are functions that translate hydrological changes into the distribution of potential economic, sociocultural and/or ecological outcomes that may arise from those changes (see product 2.7 (receptor impact modelling) for applied examples, Figure 3). Within BAs, hydrological changes are described by hydrological response variables. Hydrological response variables are defined as hydrological characteristics of the system that potentially change due to coal resource development (for example, drawdown or the annual flow volume). They are thought to be instrumental in maintaining and shaping the ecological components, processes and values provided by the ecosystems in each landscape class. Examples of hydrological response variables are found in product 2.7 (receptor impact modelling) (Figure 3). A receptor impact variable is defined as a characteristic of the system that, according to the conceptual modelling, potentially changes due to changes in hydrological response variables (for example, condition of the breeding habitat for a given species, or biomass of river red gums). Economic, sociocultural or ecological outcomes are represented by receptor impact variables, and a receptor impact model portrays the relationship between a particular receptor impact variable (e.g. the percent foliage cover of woody riparian vegetation) and one or more hydrological response variables (e.g. the change in depth to groundwater).
Receptor impact models are only developed for ecological receptor impact variables in BAs. Potential impacts on economic assets were assessed in BAs by estimating the changes to the availability of groundwater and surface water (see product 3-4 (impact and risk analysis) for details, Figure 3). Likewise, some sociocultural assets have a direct relationship with groundwater and surface water and are treated similarly to economic assets. Other sociocultural receptor impact variables may have an ecological component that can be assessed by an ecological receptor impact model.
Ecological receptor impact modelling is the focus of this submethodology: a method is provided that links hydrological response variables to receptor impact variables enabling prediction of ecological responses to coal resource development. The hydrological response variables may interact with and produce cumulative impacts on an ecological receptor. Ecological receptor impact models quantify the potential impacts on water-dependent assets that may have ecological value. In the ecological scientific literature, receptor impact models are often known as ‘ecological response functions’ (Boulton et al., 2014). This submethodology develops a novel approach to constructing ecological response functions that allows for direct, indirect and cumulative impacts, while coherently incorporating uncertainty, expert assessments and potentially empirical data.
Two potential futures are considered by BAs:
- baseline coal resource development (baseline): a future that includes all coal mines and CSG fields that are commercially producing as of December 2012
- coal resource development pathway (CRDP): a future that includes all coal mines and CSG fields that are in the baseline as well as those that are expected to begin commercial production after December 2012.
The difference in results between the CRDP and baseline is the change that is primarily reported in a BA. This change is due to the additional coal resource development – all coal mines and CSG fields, including expansions of baseline operations, that are expected to begin commercial production after December 2012. BAs focus solely on water-related impacts, and specifically those related to water quantity and availability. Potential water quality hazards and pathways are identified but any (qualitative) analysis is limited to salinity as other water quality impacts beyond the scope of BA.
Ecological receptor impact models in BAs are relevant to specific landscape classes that are potentially impacted by the additional coal resource development over the baseline, encapsulated by the CRDP. A landscape class is defined as an ecosystem with characteristics that are expected to respond similarly to changes in groundwater and/or surface water due to coal resource development (companion submethodology M03 (as listed in Table 1) for assigning receptors to water-dependent assets (O’Grady et al., 2016); companion submethodology M05 (as listed in Table 1) for developing a conceptual model of causal pathways (Henderson et al., 2016)). For each potentially impacted landscape class, the overall conceptual approach is to propagate uncertainty from hydrological models into receptor impact models (see companion submethodology M09 (as listed in Table 1) for propagating uncertainty through models (Peeters et al., 2016)). The probabilistic outputs from the hydrology models, which depend on the choice of futures (baseline or CRDP), become the inputs into the receptor impact models. The receptor impact models, in turn, produce probabilistic predictions of the receptor impact variables conditional on the hydrological inputs. This approach thus provides a coherent probabilistic assessment of the receptor impact variable while accounting for the direct, indirect and cumulative impacts of CSG and coal mining development.
The receptor impact modelling described by this submethodology is a crucial part of the cumulative impact and risk analysis process used in a BA (Component 3 and Component 4; Figure 3). Receptor impact modelling is a key step of the risk analysis as it converts the potentially abstract information about hydrological changes to ecological variables of scientific interest that stakeholders care about and can more readily understand and interpret. In particular, outcomes of the modelling will relate more closely to their ecological values and beliefs and therefore support community discussion and decision making about acceptable levels of coal resource development.
Receptor impact models may be constructed for three types of asset groups: economic, sociocultural and ecological. An overview of the construction of the receptor impact model for the three asset groups is provided in this section. The primary focus of this submethodology is on ecological assets; only ecological receptor impact models were developed. Subsequent sections concentrate entirely on ecological assets. Any subregion-specific deviations from this approach, or gaps and limitations, are addressed in product 2.7 (receptor impact modelling) for that subregion.
CRDP = coal resource development pathway, GW = groundwater, HRVs = hydrological response variables, RIVs = receptor impact variables, SW = surface water
1.1 Economic assets
In BAs, economic water-dependent assets are confined to groundwater and surface water management zones or areas and comprise specific water access entitlements or rights and other water supply features or infrastructures. Within these asset subgroups there may be many individual asset elements (e.g. water supply bores within a groundwater management zone).
The scope of the BA methodology (Barrett et al., 2013) does not require detailed analysis of the economic impacts of changes induced by the development of the coal resources. Instead the impact and risk analysis concludes at the assessment of changes to the availability of the relevant groundwater or surface water.
Within the scope of BA, potential impacts to economic assets are tied directly to potential hydrological response variables, which can be assessed against specific management thresholds, whereas indirect impacts and complex feedbacks are anticipated for the ecological assets that are the focus of this submethodology. Within BA, there is no need for economic receptor impact modelling as the range of potential hydrological change can be considered against already defined thresholds such as those specified by the NSW Aquifer Interference Policy (NSW Office of Water, 2012) or the requirements of water resource plans under Queensland’s Water Act 2000.
Economic assets are discussed no further in this submethodology but are discussed in companion submethodology M10 (as listed in Table 1) (Henderson et al., 2018) where there is a broader consideration of impacts and risks.
1.2 Sociocultural assets
In BAs, water-dependent sociocultural assets are classified into ‘Heritage site’, ‘Indigenous site’ or ‘Recreation area’ asset classes. Receptor impact modelling is not directly undertaken for sociocultural assets and the impact and risk analysis is limited to characterising the hydrological change that may be experienced by those assets. In some cases, sociocultural assets also have obvious ecological value (e.g. the health of a wetland that is also identified as having Indigenous value for fishing) and the changes to ecological receptor impact variables may be particularly important for identifying potential impacts and risks to them.
Sociocultural assets are discussed no further in this submethodology. However, it is important to note that the receptor impact model for ecological assets will also play an important role in assessing any hydrological changes for those sociocultural assets that have ecological characteristics.
1.3 Ecological assets
The construction of receptor impact models for ecological assets and ecosystems presents great challenges. The first overarching challenge is that ecological systems are complex and constantly changing. There are inherent limits to explaining patterns and to predicting outcomes for ecological variables. Logically incorporating this predictability problem into an uncertainty analysis can be practically challenging to implement. It is therefore incumbent for the approach to document and demonstrate how it will quantitatively assimilate the current state of knowledge, and use this information in such a way to ensure the applicability of the general approach to a large number of assets with various spatial footprints and at different points in time.
The second challenge for ecological receptor impact models is the specific nature of the analysis. The analysis requires assessing impacts at different points in time. However, the hydrological impacts of coal resource development in the subregion or bioregion are non-stationary, that is, the impacts vary over time because of the timing of coal resource developments, the lagged response of hydrological response variables to development pressures, and the lagged response of receptor impact variables to changes in the hydrological response variables. Thus, while it is attractive to conceptualise the problem in terms of a comparison of the existing hydrological regime before coal resource development and the new regime after coal resource development, such a simple temporal breakdown does not adequately reflect key aspects of the problem. The hydrological regime will typically change continuously as coal resource developments begin and change their patterns of water use and management during different operational phases. At the end of the coal resource development, many aspects of the hydrological regime could potentially return to their previous state or alternatively undergo a perturbed trajectory. The transitory effects may not completely be exhausted even by the end of the longest-term projection for the receptor impact variable in a BA. Thus, the problem cannot be considered as a simple change of steady state.
The non-stationarity of hydrological impact also restricts the available data that can be used to empirically estimate a receptor impact model. Fundamentally, the sequence of hydrological changes may be a key determinant of change in the receptor impact variable. However, many of the hydrological changes, both in magnitude and sequence, may be novel in these systems. Sparse data will sometimes exist to empirically calibrate these relationships. The use of ecosystem modelling is similarly restricted by the lack of process knowledge and associated data to calibrate the relationships contained in the model. Techniques such as Bayes Nets could be considered (e.g. Marcot et al. (2001)), but they do not naturally accommodate spatial and temporal phenomena, and entail significant resources to parameterise.
The fractured nature of the knowledge base means that the use of expert opinion will typically be needed to construct receptor impact models, which can be assessed and updated with empirical data where possible. The experts can integrate their knowledge base to make predictions about likely outcomes related to hydrological change (O’Hagan, 1998). Carefully constructed questions help experts focus on the key issues that need to be considered and elicit their response in a structured and transparent way.
The elicitation process requires careful construction (O’Hagan et al., 2006). It is well known that poorly designed elicitations can seriously impact on the reliability of the results. Ambiguous questions mean that experts may misunderstand the task and introduce additional uncertainty into the analysis. Poor processes can lead to problems, such as dominance of debate by vocal individuals. Inadequate protocols can lead to issues, such as anchoring, where experts do not explore the full extent of their knowledge and beliefs. Unorganised, inefficient or unclear protocols can confuse experts and lead to burn out or decreased motivation, which leads them to drop out from the elicitation process. There is also a balance between choosing a protocol that is simple to explain versus a complex protocol that requires substantial training and time commitment of experts. A very pragmatic protocol is one that allows experts to contribute their knowledge early without too much education on the elicitation process. On the other hand, overly simplistic protocols may not elicit the experts’ knowledge correctly and can also lead to confusion on the nature of what exactly was elicited if the education portion of the elicitation process is reduced too much. These trade-offs are of particular importance for receptor impact models deployed in a BA, where many such models are developed across a subregion or bioregion and the demand for expert involvement and motivated participation is essential for successful completion of each task. Ideally, the same experts will be involved for deriving conceptual models and also the quantitative probabilistic receptor impact models. But the method described here also allows for the pragmatic cases where different experts contribute at various times, for example, because of availability limitations or a shift in the focus of domain expertise for a given landscape class.
The opinions expressed by different experts will sometimes disagree. This fact simply reflects variation in their understandings and beliefs and so does not directly undermine the use of the approach. However, it means that the choice of experts can have a material effect on the analysis, so the elicitation needs to be done in a consistent, flexible and principled way. In some senses, the experts operate as a jury. Provided the experts represent the diverse views across the relevant informed community within their consensus opinion, readers will have confidence that a wide range of informed opinions have been considered and reported in the Assessment. The choice of experts, including their identification, invitation and participation in the process, needs close attention to ensure appropriate expertise is included. In practice, expert availability can be a non-trivial constraint. The expert invitation process was a collaborative effort among the Office of Water Science, the Bureau of Meteorology and the BA ecology discipline teams for each bioregion or subregion, which provided communication channels to key regional institutions and individuals with a wide range of expertise.
Ultimately, however, the best way to assess the experts’ judgement is to collect relevant empirical data wherever possible. Experts will be encouraged to include knowledge of existing empirical data from independent sources within their assessments. A very important additional objective of the receptor impact model approach is to allow for the coherent incorporation of relevant empirical data if it were to become available. These data may be obtained through a defined monitoring program that targets a receptor impact variable as part of validating (or invalidating) the risk predictions and the characterisation of the receptor impact model. This goal for coherent data assimilation dictates the choice of model structure, which must allow for potentially very different forms of ecological data, such as continuous (e.g. abundance), non-negative integers (e.g. counts), binomial counts (e.g. percent cover) or binary (e.g. presence–absence) responses. This goal, which seeks to ensure the coherent updating of uncertainty estimates given potential empirical data, also guides the selection of the receptor impact variable, where it forms a clear criterion that any selected receptor impact variable must at least in principle be measurable. Such a requirement additionally ensures that the target receptor impact variable is both well-defined and also accessible to expert assessment.
In summary, the risks in the use of expert-based information can be managed by appropriate protocols and procedures. The workflow for ecological receptor impact modelling is outlined in Figure 4. Input from independent external ecology experts contributes to the workflow at three separate stages, as does hydrological modelling simulation output and the expertise of the BA hydrology modellers. Both the external experts and the hydrologists contribute to the selection of hydrological response variables that are ecologically meaningful and also amenable to hydrology modelling. The careful definition of landscape class forms the spatial context for the expert elicitation and receptor impact model analysis (Chapter 2). Experts should be engaged in a structured way with strong facilitation to ensure clarity of communication and focus on the issue in question. Elucidation of the ecological ecosystem within a landscape class is conducted at a qualitative modelling workshop that maps the key ecological variables, hydrological response variables and the linkages among these variables (Chapter 3). This modelling exercise is used to choose key hydrological response variables and receptor impact variables to progress for a receptor impact model analysis (Chapter 4). Based on the selected hydrological response variables and receptor impact variables, an efficient design of elicitation scenarios is constructed, which directly addresses the resource and time limitations imposed by expert availability (Chapter 5). The expert education and elicitation process as experienced by the experts and its theoretical underpinning is given in Chapter 6. The derivation of the receptor impact model and prediction methods are given in Chapter 7 and Chapter 8. Each stage is described in the following subsections.
The workflow leads to the construction of a receptor impact model (RIM) that predicts the response of a receptor impact variable (RIV) conditional on hydrological response variables (HRVs). The uncertainty encapsulated by the hydrology modelling is propagated through the RIM when predicting the RIV response to the choice of BA futures (baseline or coal resource development pathway) across a landscape class. Workshop steps are shown in red, ecology and hydrology expert input sources are shown in blue.
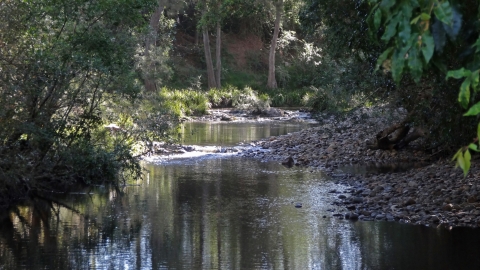
METHODOLOGY FINALISATION DATE
- 1 Background and context
- 2 Identification of potentially impacted landscape classes (stage 1)
- 3 Qualitative mathematical modelling (stage 2)
- 4 Identification of hydrological response variables and receptor impact variables (stage 3)
- 5 Development of scenarios for receptor impact model expert elicitation (stage 4)
- 6 Receptor impact modelling workshop (stage 5)
- 7 Receptor impact model estimation (stage 6)
- 8 Receptor impact model prediction (stage 7)
- 9 Content for product 2.7 (receptor impact modelling)
- References
- Citation
- Acknowledgements
- Contributors to the Technical Programme
- About this submethodology