- Home
- Assessments
- Bioregional Assessment Program
- Galilee subregion
- 5 Outcome synthesis for the Galilee subregion
- What are the potential impacts of additional coal resource development on ecosystems?
The impact and risk analysis (Lewis et al., 2018) used multiple lines of evidence (Box 7) to investigate how hydrological changes due to additional coal resource development may affect ecosystems in the assessment extent.
These ecosystems are represented by 31 landscape classes (Evans et al., 2018b), which were further aggregated into 11 (bolded) landscape groups (Box 8):
- ‘Dryland’, includes vegetation that depends only on incident rainfall and localised runoff, and so is not considered to be water dependent for purposes of this assessment
- Floodplain environments, include wetlands that are dependent on groundwater (‘Floodplain, wetland GDE’), wetlands that are disconnected from groundwater (‘Floodplain, disconnected wetland’) and vegetation outside of wetlands that is groundwater dependent (‘Floodplain, terrestrial GDE’) or surface water dependent (‘Floodplain, non-wetland’)
- Non-floodplain environments, include wetlands that are dependent on groundwater (‘Non-floodplain, wetland GDE’), wetlands that are disconnected from groundwater (‘Non-floodplain, disconnected wetland’), and vegetation outside of wetlands that is groundwater or surface water dependent (‘Non-floodplain, terrestrial GDE’)
- Streams, include streams that are dependent on groundwater (‘Streams, GDE’) and streams that are disconnected from the groundwater system (‘Streams, non-GDE’). Streams in each landscape group are classified based on water regime (temporary or near-permanent) and landscape position (lowland, upland or estuarine)
- ‘Springs’, include both discharge springs and recharge springs.
Potential impacts on ecosystems were assessed by first overlaying their extent on the zone of potential hydrological change (Box 5, Figure 13). Assessing potential impacts for the ‘Springs’ landscape group also used other drawdown zones relevant to individual spring source aquifers (such as the Clematis Group aquifer for the Doongmabulla Springs complex).
For the potentially impacted ecosystems within the zone (Table 2), receptor impact modelling (Box 9) was used to translate predicted changes in hydrology into a distribution of ecological outcomes that may arise from those changes. These models used indicators of the health of the ecosystem, such as the annual percent foliage cover for selected tree species, to infer the potential ecological impacts of hydrological changes.
The relative risk to ecosystems is reported using categories defined in Box 10.
Potential impacts to water-dependent ecosystems and assets were assessed using multiple lines of evidence that included: Impacts to all assets and ecosystems were assessed (as a minimum) by overlaying the extent of ecosystems and assets on the zone of potential hydrological change to identify the hydrological changes that a particular asset or ecosystem might experience. For ecological assets, the assessment considered the potential impact to the habitat of species, not to the species themselves. Ecosystems and assets that fall within the mine exclusion zone are likely to be impacted, but as estimates of drawdown are unreliable, quantification of the impact is not possible. Similarly, surface water modelling near mine pits cannot quantify the impact on some streams. The greatest confidence in results is in those areas that are very unlikely to be impacted. Where potential impacts have been identified, further local-scale modelling using higher resolution data may be required to determine the presence and magnitude of impacts. Box 7 Analysing impact and risk
Box 8 Understanding the landscape classification
The natural and human-modified ecosystems in the subregion were classified into 31 landscape classes (Section 3.4 in Lewis et al. (2018)) to enable a systematic and comprehensive analysis of potential impacts on, and risks to, the water-dependent assets nominated by the community. The landscape classification was based on the subregion’s geology, geomorphology, hydrogeology, land use and ecology. These landscape classes were aggregated into 11 landscape groups based on their likely response to hydrological changes. Definitions for landscape classes and landscape groups for the Galilee subregion are available online at environment.data.gov.au/def/ba/landscape-classification/galilee-subregion.
About 6% of the zone of potential hydrological change, along the far eastern edges, does not have landscape classes or groups mapped, as this part of the zone extends beyond the margins of the assessment extent. This recognised limitation may mean that specific results reported in this synthesis for potentially impacted areas, lengths and proportions of some ecosystems may be slightly underestimated or overestimated. Despite this minor limitation, the main findings of the landscape group analysis are unlikely to be substantially different, as most ecosystems in the zone are well mapped (particularly in areas predicted to experience the greatest hydrological changes), and the majority of the unmapped area is likely to be ‘Dryland’ or ‘Floodplain, non-wetland’ ecosystems, both of which do not rely on access to groundwater or streams.
Box 9 Receptor impact models
Receptor impact models translate predicted changes in hydrology into ecological outcomes that may arise from those changes. Applying receptor impact models across ecosystems assists in identifying where changes in hydrology may result in ecosystem changes, and consequently where additional local-scale information and further investigation may be warranted.
To assess potential ecological outcomes experts identified receptor impact variables, characteristics that serve as indicators of the ecological condition of an ecosystem, and which are also likely to respond to hydrological changes as well as being within the expertise of the available experts. These variables were specifically chosen to be representative of a landscape group in the Galilee subregion and include the following indicators:
- percent foliage cover of woody riparian vegetation
- density of a mayfly species (nymphs), 3 months after the end of the wet season
- percent foliage cover of woody vegetation.
One or more hydrological response variable(s) that affect each indicator were identified with ecological experts. Receptor impact models, which take the form of statistical models, were then developed to represent the relationship between each indicator and its important hydrological response variables. Details are found in Ickowicz et al. (2018).
Hydrological models were used to quantify changes in the related hydrological response variable(s). Predictions of an ecological indicator at a specific location were made by applying the receptor impact model for that indicator to the predicted hydrological response variable(s) at that location.
Receptor impact models were used to predict changes in the indicator for a landscape group that result from changes in the hydrological response variable(s). The changes in the indicator reflect the magnitude of potential ecological impacts for that ecosystem. The indicators provide a relative measure of the risk to the ecosystem, rather than a prediction about (for example) densities of mayfly nymphs per se.
For the Galilee subregion, the impact and risk analysis focused on ecosystems represented by five landscape groups. Four receptor impact models were developed, representing four of the landscape groups. In addition, 12 qualitative mathematical models were developed for the five landscape groups. The ‘Springs’ landscape group only had qualitative mathematical models built, as it was not possible to develop a receptor impact model. Results from applying receptor impact models are described in Section 3.4 of Lewis et al. (2018).
Importantly, receptor impact models were not used in isolation but were applied along with other lines of available evidence, including expert advice, hydrological modelling results and other existing data and knowledge, to assess potential ecological impacts.
Box 10 Categorising risk for ecosystems
Parts of some ecosystems were deemed at greater risk of ecological and hydrological changes relative to other parts of that ecosystem. Three categories were defined: ‘more at risk of ecological and hydrological changes’, ‘at some risk of ecological and hydrological changes’ and ‘at minimal risk of ecological and hydrological changes’. Categorisation assists the rule-out process and in identifying where further local-scale assessment is warranted.
Assessment units that overlap with a landscape group or class are categorised based on the degree that modelled ecological changes exceed thresholds of risk. These bioregion-specific thresholds are based on expert opinion and defined using receptor impact variables listed in Box 9 (see Section 3.4 of Lewis et al. (2018) for more details on the thresholds).
Table 2 Extent of each landscape group in the Galilee assessment extent and the zone of potential hydrological change
The extent of each landscape group is either an area of vegetation (km2), length of streams (km) or number of springs. The landscape groups that have qualitative and/or receptor impact models are also shown.
Definitions for landscape classes and landscape groups for the Galilee subregion are available online at
environment.data.gov.au/def/ba/landscape-classification/galilee-subregion.
Due to rounding, some totals may not equal the sum of the individual numbers.
GDE = groundwater-dependent ecosystem
Data: Bioregional Assessment Programme (Dataset 1, Dataset 15)
Figure 13 Landscape groups within the zone of potential hydrological change
The coal resource development pathway (CRDP) includes baseline and additional coal resource developments (ACRD). GDE = groundwater-dependent ecosystem
Data: Bioregional Assessment Programme (Dataset 12, Dataset 15)
Ecosystems
Which ecosystems are very unlikely to be impacted?
Ecosystems outside the zone of potential hydrological change are very unlikely to be impacted, including:
- 526,658 km2 of remnant vegetation and 71,563 km2 of non-remnant vegetation. This includes 101,460 km2 of groundwater-dependent vegetation and 20,373 km2 of wetland vegetation
- 387,170 km of streams that are groundwater dependent and 341,433 km of streams that are not groundwater dependent. Streams outside of the zone predominantly have an intermittent or ephemeral water regime (386,468 km) rather than a perennial or near-perennial water regime (552 km)
- 8133 km2 of saline wetlands and 290 km of estuarine streams that cover less than 0.1% of the assessment extent.
Within the zone, most (73%) of the ecosystems are categorised as dryland vegetation (8134 km2) or floodplains not connected to wetlands (2098 km2) (Table 2 and Figure 13). These are ruled out as they depend on incident rainfall and localised runoff, rather than groundwater or streams.
It is very unlikely that the source aquifers of any of the Great Artesian Basin springs in the Eromanga Basin will be impacted by the modelled additional coal resource developments. Key finding 8
Assessing potential impacts to springs uses drawdown zones relevant to the source aquifer for each spring, and these may differ from the zone of potential hydrological change (which relates to the near-surface aquifer). Several spring clusters in the zone defined by the near-surface aquifer, including the Doongmabulla Springs complex, rely on groundwater sourced from deeper (confined) aquifers of the Clematis Group and upper Permian coal measures.
Using the relevant drawdown zones for different spring groups in the Galilee assessment extent shows that 1359 springs are very unlikely to be impacted due to modelled additional coal resource development. This includes 6 springs in the Doongmabulla Springs complex and 1353 GAB springs that are sourced from aquifers of the Eromanga Basin (which partly overlies the older Galilee Basin, Figure 5 and Figure 6). Potential impacts to the GAB (Eromanga) springs, which occur over 50 km west of the seven mines, are ruled out as the groundwater modelling for this assessment indicated that drawdown due to modelled additional coal resource development will not affect any aquifers of the Eromanga Basin (Peeters et al., 2018). This is due to the very thick Moolayember Formation (400 to 1000 m thick), a low permeability regional aquitard layer, that separates the Eromanga Basin aquifers from the underlying Clematis Group aquifer (in areas where greater than 0.2 m drawdown in the Clematis Group is very unlikely).
Which ecosystems are potentially impacted?
Ecosystems that intersect the zone of potential hydrological change (Box 5, Figure 13) are potentially at risk of impact due to additional coal resource development (Table 2, Figure 13).
At the Doongmabulla Springs complex near the Carmichael River, there is a 5% chance that additional drawdown in the Clematis Group source aquifer will exceed 0.2 m for 181 of 187 springs. Thus, potential ecological impacts cannot be ruled out, although local-scale information is needed to improve the understanding of impacts to these springs. It is very likely that at least five of the seven springs sourced from the upper Permian coal measures will be affected by greater than 5 m of additional drawdown. Key finding 9
Springs
Three clusters of springs are within the zone: the Doongmabulla Springs complex, Permian springs cluster and Triassic springs cluster. Springs are not explicitly incorporated in the groundwater model, with drawdown at each spring estimated for the model layer interpreted as the source aquifer (e.g. Clematis Group aquifer). However, the regional-scale modelling in this assessment is not well suited to accurate point-scale drawdown estimates, such as for individual springs. This means that more detailed modelling user higher resolution local-scale data is necessary to better understand impacts on springs.
The available hydrogeological evidence to characterise the source aquifers for the springs within the zone is discussed in Section 3.4 of Lewis et al. (2018). The Permian springs are sourced from the upper Permian coal measures (layer 5 in the Galilee groundwater model), whereas the Triassic springs are ‘recharge springs’ disconnected from the regional groundwater system and likely sourced from local sedimentary rock aquifers of Triassic age (such as the Dunda beds, which are not included in the model).
As outlined in Section 3.4.3.1 of Lewis et al. (2018), two different interpretations have been presented by previous researchers for the source aquifer of the Doongmabulla Springs complex; one interpretation favours the Clematis Group as the primary source aquifer, whereas the other proposes the upper Permian coal measures. Appraisal of the available hydrogeological evidence for this assessment, including the regional groundwater flow directions of different aquifers and the topographic location of the springs, suggests that most of the Doongmabulla Springs complex is sourced from the Clematis Group. This means that the drawdown predictions from the Galilee groundwater model for the Clematis Group layer can be used to estimate potential groundwater impacts at Doongmabulla.
The geological structure used in the groundwater model, and the way in which the proposed coal mine operations may affect groundwater levels for each modelled aquifer, was developed to provide the greatest utility and spatial coverage to define the zone of potential hydrological change. However, there are some areas in the zone where both the model structure and the effects of mine dewatering will potentially cause the amount of drawdown to be over-estimated (see Section 3.3 in Lewis et al. (2018) for further information). This will mainly affect areas where the Quaternary alluvium and Cenozoic sediment layer does not occur, such as in the upland regions west of the proposed mines in the zone.
To better understand the likely overestimation of drawdown in these areas, an alternative groundwater model conceptualisation was used to predict drawdown impacts at some specific points. This alternative model provides more robust drawdown predictions in areas of outcropping Triassic rocks in the zone, particularly for the Clematis Group aquifer. The variations between the two model conceptualisations, including discussion of why the alternative approach is more scientifically valid for making drawdown predictions at the Doongmabulla Springs complex, is outlined in Box 3 and explained in detail in Lewis et al. (2018) and Peeters et al. (2018).
The alternative model predictions are better suited to evaluating drawdown for the Doongmabulla Springs complex, and these generally project lower levels of drawdown for the springs than the results from the original groundwater conceptualisation. For example, the drawdown estimated at one of the springs in the Doongmabulla Springs complex is 0.88 m (with a 50% chance) using the original conceptualisation, but is 0.18 m using the alternative approach. Although model results from both of the conceptualisations indicate that 181 of the 187 individual springs in the Doongmabulla Springs complex have at least a 5% chance of more than 0.2 m of drawdown, there are no springs with a 50% chance of exceeding 0.2 m drawdown under the alternative conceptualisation (Table 20 in Lewis et al. (2018)).
Estimates of expected water level reductions due to modelled additional coal resource development within the source aquifers to the various springs indicate that there is some potential for ecosystem impact. This was investigated in this assessment using qualitative models to show the ecological relationships within aquatic communities (see Section 2.7.3 in Ickowicz et al. (2018)). The following ecosystem impacts for the different spring clusters were predicted:
- Doongmabulla Springs complex: Changes in water flows and a decrease in water availability to GDEs are predicted; however, the long-term impact on the springs and spring wetlands and related organisms is unclear (Figure 14, and Section 3.4.3.3 in Lewis et al. (2018)).
- Triassic springs cluster: Drawdown for the 12 springs in the Triassic springs cluster cannot be reliably estimated by the groundwater model used in this assessment, but is likely to fall within the range predicted for the Clematis Group model layer (Figure 14, and Section 3.4.3.3 in Lewis et al. (2018)).
- Permian springs cluster: It is very likely that at least five springs and very unlikely that more than seven springs will experience drawdown in excess of 5 m in the upper Permian coal measures due to modelled additional coal resource development. Potentially affected springs include the Albro, Lignum, Storys and Mellaluka springs. Hydrological changes will potentially result in the loss of ecological functioning of these springs (Figure 14, and Section 3.4.3.3 in Lewis et al. (2018)).
The groundwater component of the zone of potential hydrological change (brown line) was defined by drawdown in the near-surface aquifer (see Box 5). Springs, however, may source water from different deeper layers. Being in or out of the zone defined by the near-surface aquifer therefore does not necessarily signify impacts – rather potential impacts are indicated by drawdown in the relevant source aquifer for that spring. Additional drawdown is the maximum difference in drawdown under the coal resource development pathway and the baseline (Box 1 and Box 4). Because there are no coal resource developments in the baseline for the Galilee subregion, the CRDP includes only the additional coal resource development (ACRD). Results are shown as percent chance of exceeding drawdown thresholds (Box 6). These appear in Lewis et al. (2018) as percentiles.
Data: Queensland Herbarium (Dataset 16); Bioregional Assessment Programme (Dataset 17)
Groundwater-dependent streams
The Galilee subregion includes the headwaters of six major surface water catchments: Cooper Creek-Bulloo, Diamantina-Georgina, Flinders-Norman, Darling, Burdekin and Fitzroy (Figure 2). About 12% of all streams in the assessment extent are groundwater dependent (Table 19 in Lewis et al. (2018)).
Almost half (2801 km) of the 6285 km of streams in the zone of potential hydrological change are groundwater dependent (Table 2) and most have a temporary water regime (2541 km of these 2801 km).
Potential hydrological impacts to groundwater-dependent streams include additional drawdown in excess of 5 m, an increase in the number of low-flow days (averaged over 30 years), increased low-flow spells and decreased overbank flows.
Key finding 10
There is some level of risk of ecological and hydrological changes in 8% of the 2801 km of groundwater-dependent streams in the zone of potential hydrological change. This includes parts of Native Companion, North and Sandy creeks, and the Belyando and Carmichael rivers.
This is based on expert opinion, modelled hydrological changes and changes to the chosen ecological indicators (Box 9); more detail in shown in Figure 51 in Lewis et al. (2018).
Temporary, upland groundwater-dependent streams are located along the western edge of the zone, upstream of the proposed Hyde Park and China Stone mines in the north, and upstream of the proposed Kevin’s Corner, Alpha and South Galilee mines in the south. Temporary, lowland groundwater-dependent streams intersect and flow downstream of the seven proposed mines in the northern and southern parts of the zone of potential hydrological change. It is very unlikely that additional drawdown in excess of 0.2 m will affect more than 1597 km of temporary, lowland groundwater-dependent streams and 466 km of temporary, upland groundwater-dependent streams (Figure 45 and Table 21 of Lewis et al. (2018)). Additional drawdown in excess of 5 m is very unlikely to affect more than 173 km of groundwater-dependent streams.
Modelled changes in the flow regime of the Belyando River below Sandy Creek and the Suttor River below the confluence with the Belyando River do not appear to lead to hydrological changes that are considered to put these streams and their ecosystems at risk.
Non-groundwater-dependent streams
The remaining streams in the zone of potential hydrological change are not groundwater dependent (3484 km) and so are unlikely to be affected by groundwater drawdown (Table 2). This includes most of the temporary streams (1028 km) in the zone that are potentially impacted but not represented in the surface water model (due to absence of model nodes on these streams), including parts of Bully, Lagoon, North, Sandy and Tomahawk creeks and Carmichael River. Future opportunities to improve the surface water modelling, which include increasing the density of modelling locations on the stream network, are further explained in the ‘Building on this assessment’ section.
Potential hydrological changes include increased number of low-flow days (averaged over 30 years) and low-flow spells along up to 177 km of temporary streams in the zone. The analysis of potential ecosystem impacts indicates that less than 1% of non-groundwater-dependent streams are ‘at some risk of ecological and hydrological changes’ (relative to other streams in the zone) (Box 10) (Figure 56 in Lewis et al. (2018)). These potential changes mainly affect isolated segments of the Belyando and Suttor rivers, just upstream of Lake Dalrymple.
Floodplain, terrestrial groundwater-dependent ecosystems
On floodplains, 3% of the 2433 km2 of groundwater-dependent vegetation is ‘at some risk of ecological and hydrological changes’ due to additional drawdown and decreased overbank flow along parts of Alpha, North, Sandy and Tallarenha creeks, and the Belyando and Carmichael rivers. Outside of floodplains or wetlands, 5% of the 1189 km2 of groundwater-dependent vegetation is ‘at some risk of ecological and hydrological changes’. These areas are near the proposed coal mines, where additional drawdown is greatest. Key finding 11
Floodplain, terrestrial GDEs typically occur in areas that are water limited, with low annual rainfall and high evaporation rates (Evans et al., 2014a). In this subregion, most groundwater-dependent remnant vegetation in the zone of potential hydrological change occurs on floodplains (2358 km2 or 62% of groundwater-dependent vegetation in the zone). Vegetation classified as ‘Floodplain, terrestrial GDE’ is located along the western edge of the zone, upstream of the proposed Hyde Park and China Stone mines in the north and upstream of the proposed Kevin’s Corner, Alpha and South Galilee mines in the south (Figure 58 in Lewis et al. (2018)).
More than half of the groundwater-dependent vegetation (716 km2) in the zone is located on floodplains intersected by temporary streams that are potentially impacted but not represented in the surface water model. Potential hydrological changes include decreased overbank flows that may affect up to 355 km2 of floodplain vegetation and additional drawdown in excess of 5 m that may affect up to 296 km2 of groundwater-dependent vegetation on floodplains. Within large uncertainty bounds, up to 3% of groundwater-dependent vegetation on floodplains is ‘at some risk of hydrological and ecological changes’ due to additional drawdown and decreased overbank floods along parts of Alpha, North, Sandy and Tallarenha creeks, and the Belyando and Carmichael rivers (Figure 61 in Lewis et al. (2018)).
Non-floodplain, terrestrial groundwater-dependent ecosystems
About one-third of GDEs in the zone (1189 km2) rely on groundwater associated with clay plains, loamy and sandy plains, inland dunefields, and fine-grained and coarse-grained sedimentary rocks. Additional drawdown in excess of 5 m is very unlikely to affect more than 68 km2 (2%) of GDEs that do not occur on floodplains in the zone. As this landscape group is not associated with streams, it is unaffected by changes to surface water flow. Up to 5% of non-floodplain terrestrial GDEs in the zone are ‘at some risk of ecological and hydrological changes’, and these occur where additional drawdown is greatest (Figure 65 in Lewis et al. (2018)).
Explore potential impacts on ecosystems in more detail on the BA Explorer, available at www.bioregionalassessments.gov.au/explorer/GAL/landscapes Conceptual modelling, product 2.3 (Evans et al., 2018b) Receptor impact modelling, product 2.7 (Ickowicz et al., 2018) Impact and risk analysis, product 3-4 (Lewis et al., 2018) Receptor impact modelling, submethodology M08 (Hosack et al., 2018) Impacts and risks, submethodology M10 (Henderson et al., 2018) Summary of groundwater drawdown by assessment unit (Dataset 1) Landscape classification (Dataset 15) Landscape class spatial overlay by assessment unit (Dataset 1) Receptor impact model (Dataset 18) Results from applying receptor impact models (Dataset 19) FIND MORE INFORMATION
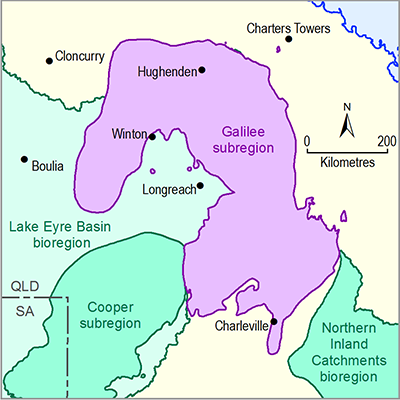
Product Finalisation date
- Executive summary
- Explore this assessment
- About the subregion
- How could coal resource development result in hydrological changes?
- What are the potential hydrological changes?
- What are the potential impacts of additional coal resource development on ecosystems?
- What are the potential impacts of additional coal resource development on water-dependent assets?
- How to use this assessment
- Building on this assessment
- References and further reading
- Datasets
- Contributors to the Technical Programme
- Acknowledgements
- Citation