The geological history of the Cooper subregion extends from the Cambrian through to the present. The Thomson Orogen and Warburton Basin were variably deformed during the Delamerian Orogeny, with metamorphic grade increasing from the relatively unaffected Warburton Basin, to the east and north-east into the Thomson Orogen. This was followed by north-west and south-east oriented compression and uplift in the Devonian to Carboniferous Alice Springs Orogeny, which resulted in the characteristic structural grain of the Cooper Basin. Thrusting of the Warburton Basin sequence formed significant ridges providing up to 600 m relief prior to deposition of the Cooper Basin sequence (Champion et al., 2009).
Basement rocks under the south-western part of the subregion experienced mega bolide impacts, which resulted in shock metamorphism and crustal rebound as well as hydrothermal alteration in rocks of the Warburton Basin (Glikson, 2013; Radke, 2009). Ridges developed as a result of north-west-directed thrust faulting in the Warburton Basin during the Alice Springs and Kanimblan orogenies, creating a high-relief paleotopography that controlled subsequent Cooper Basin sedimentary deposition in the main three troughs (Gravestock and Jensen-Schmidt, 1998; Raymond et al., 2012).
Granite bodies were emplaced in the subregion at two periods prior to deposition of the Cooper Basin rocks. An arcuate granite belt beneath the southern part of the subregion in Queensland and SA was emplaced during the Early Devonian, while granites beneath the Nappamerri Trough, including the Big Lake Suite granodiorite, were emplaced in the middle Carboniferous and earliest Permian (Gatehouse et al., 1995).
The Cooper Basin represents Permian to Triassic deposition which finished at the end of the Early Triassic with regional uplift, tilting and erosion. The basin contains a number of stacked non-marine depositional sequences that are contained within the Late Carboniferous to late Permian Gidgealpa Group and the late Permian to Early Triassic Nappamerri Group (Jensen-Schmidt et al., 2006).
The early depositional history of the Cooper Basin was dominated by glaciers moving across a landscape influenced by major basement ridges. The terminal stages of glaciation were marked by widespread peat swamp deposition (Patchawarra Formation). This was followed by relative tectonic and structural stability through most of the early Permian, which is reflected by lacustrine deposits (Murteree and Roseneath shales) (Draper, 2002b; Gravestock and Jensen-Schmidt, 1998).
Structural growth of the basement ridges commenced in the early Permian, coinciding with deposition of the Epsilon Formation, and culminated in the Daralingie unconformity, a tectonic event that created significant erosion on some ridges (Moussavi-Harami, 1996). The erosional effects of the Daralingie Unconformity are more severe in the north-eastern Cooper Basin in Queensland where the late Permian Toolachee Formation disconformably overlies the early Permian Patchawarra Formation with intervening units absent. Concomitant with this uplift, reverse faulting has been attributed to renewed compression and deformation of the eastern Australian marginal basins (Draper, 2002b; Gravestock and Jensen-Schmidt, 1998).
The Toolachee and Arrabury formations were deposited during a period of tectonic stability. Tectonism recommenced during deposition of the Tinchoo Formation, resulting in north-west tilting of the Cooper Basin, which is reflected in a northerly thickening of the Nappamerri Group, and erosion from the southern areas of the basin (Simon, 2000). Regional uplift and erosion terminate deposition in the Cooper Basin at the end of the Middle Triassic (Jensen-Schmidt et al., 2006).
The Eromanga Basin represents a series of marine transgressions and regressions during the Jurassic and Cretaceous. From the Early Jurassic through to Early Cretaceous periods, the lower non-marine succession accumulated through large sand-dominated braided fluvial systems that drained centripetally into lowland lakes and swamps. This was during a period of tectonic stability with subsidence being the major mechanism accommodating deposition. Sediment coverage was relatively thin, continuous and widespread during this time, and thickest within the Central Eromanga Depocentre (Ransley et al., 2012a).
Subduction and associated volcanism occurred concurrently on the eastern margin of the Australian Plate, on the eastern Australian volcanic arc. Consequently sediment was contributed from two sides of the depocentre. A cratonic source contributed clean quartzose sands from the south-west, whereas volcanogenic detritus came from the volcanic province along the eastern subduction zone (Ransley et al., 2012a).
Several multiple bolide impacts occurred during deposition of the Cadna-owie Formation (Glikson, 2013). Following this, during the Early Cretaceous, there was extensive marine inundation over large areas of the Australian continent. There was a period of rapid subsidence between 112 and 99 Ma. As a result, thick marine shales accumulated with interspersed thin sandstone units during minor regressive cycles (Ransley et al., 2012a).
With uplift of the eastern highlands commencing in the Late Cretaceous, erosion of this uplifted region contributed sediment for the rapid accumulation within the Eromanga Basin that swamped the marine conditions. This led to accumulation of an upper non-marine succession from meandering fluvial systems that crossed a floodbasin dominated by coal swamps and lakes (Winton Formation). The deposition of the upper non-marine sequence contributed almost half the total sediment thickness of the Eromanga Basin. Erosion and deep weathering commenced during the Late Cretaceous and Paleocene (Ransley et al., 2012a).
The Lake Eyre Basin represents terrestrial deposition punctuated by tectonic activity and deep weathering. Major anticlines, including the Innamincka Dome, formed as a result of compression related folding in the Oligocene. This was followed by compressional folding and faulting during the Miocene, reflected in domed and faulted silcrete horizons. Significant uplift of between 350 and 500 m has been reported within the Cooper subregion (Ransley et al., 2012a).
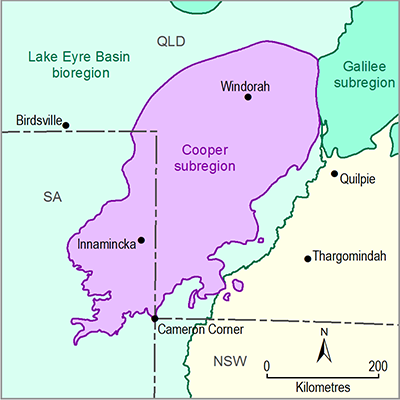