- Home
- Assessments
- Bioregional Assessment Program
- Methods
- Bioregional assessment methodology
- 5 Impact and risk analysis
5.1 Demonstrated reliance on water either directly or indirectly
Water-dependent assets have a demonstrated reliance on water, either directly or indirectly, and are likely to be exposed to change in water quantity or quality through either coal mining or CSG extraction. There are three ways in which this reliance can occur:
- Identified assets may be directly or indirectly and materially affected by exposure to changes in water quality or quantity from CSG or coal mining. For example:
- Assets and receptors may be directly affected by exposure to changes in water quality or quantity through permanent or ephemeral streams or water bodies; through overland flow or subterranean aquifer discharges; or (in the case of stygofauna) through subterranean aquifers. A substantial number of nominally aquatic species rely on access to open or flowing water for part or the majority of their life cycles. This group includes fish, frogs, turtles, crocodiles, many birds, migratory species, invertebrates, other biota and a range of mammal species and ecological processes, plus a large number of plants that grow submerged or semi-submerged in water bodies or require permanent or seasonal inundation in swamp and marsh habitats. Many of these species, or the assemblages they form, also have cultural associations for both Indigenous and non-Indigenous Australians, including waterbirds (such as magpie geese), turtles, crocodiles, platypus and fish (such as eels and barramundi). Recognition of these associations is paramount to Indigenous people, but also to many non-Indigenous people, for whom the health of their environment is measured through the presence of fish in rivers and birds on lakes. Consequently, existing environmental legislation not only recognises species and communities that are threatened at the local, national or international scale, but also protects many aquatic environments by limiting activities that may be directly harmful to them. Groundwater availability is also important at drier times of year for the persistence of many natural communities and some agricultural systems.
- Assets and receptors may be indirectly affected by exposure to changes in water quality and quantity through material reliance on another species or community that have been directly affected by exposure to changes in water quality and quantity. For example, the majority of plant assemblages in Australia depend on groundwater to survive drier parts of the year, and associated fauna require their persistence to provide shelter and food. Other animals that are not considered aquatic may rely on aquatic ecosystems for feeding, breeding or shelter at particular times of the year. Such animals may include domestic stock in extensive (rangeland) contexts, or where watering points are on-stream.
- For some identified assets, impacts are potentially chronic rather than acute. Rapid changes in population size or structure may result from acute impacts on water quality or quantity. Less marked – but equally significant – chronic impacts can affect populations over time through chronic exposure to change with repeated but subtle effects on reproductive success, dispersal, succession, predation, etc. Assets affected through exposure to chronic impacts include species and communities, but also processes such as aquifer recharge. Agricultural assets may be exposed through changes in water quality or availability for irrigation.
- Reversible or irreversible receptor and asset responses. Some direct, indirect and cumulative impacts may be considered irreversible (for example, subsidence impacts on water flows in groundwater or surface water systems). These impacts may be positive or negative in nature and may cease when depressurisation stops or continues. Other irreversible impacts may include species extinction, invasive species or transgression beyond an ecosystem 'tipping point'. As such, all impacts must be considered as reversible or irreversible and reported in the BA.
Some identified assets may not be materially affected by changes in water quality or quantity if they have low dependence on groundwater or obtain their water from other areas, such as direct or occult precipitation. These assets should be recorded in the workflow but not considered further in the analysis.
5.1.1 Tractability for measuring or monitoring
Coal mining or CSG extraction may lead to water quality and quantity impacts that are spread over space and time. As a result, a range of anthropogenic and ecological assets may be affected, and those changes may be perceived to be either positive or negative depending on context, timing or perspective. For those assets that have been clearly demonstrated to rely on groundwater, the potential to measure or monitor them needs to be determined, as without adequate baseline measurement or ongoing monitoring, the magnitude and direction of change is likely to be uncertain and possibly contentious. A series of levels of tractability exist, from the highly measurable to the unmonitorable.
Some receptors of certain asset classes are likely to be highly measurable and monitorable. The following asset classes are likely to be sufficiently well understood in a spatial and temporal context that they can be accurately and repeatedly measured:
- processes, such as within-bank flow
- environmental metrics, such as water quality
- agricultural assets
- ecological assets, such as fish populations and waterbird breeding success.
Some receptors are likely to be measurable but not tractable to monitor. These may include receptors that are very difficult to adequately sample. For instance, samples of deep groundwater may be analysed for stygofauna content, which would allow a measurement of species diversity and abundance, but with limited understanding of the ecology and distribution of various stygofaunal groups. Monitoring change – and drivers of change – may be logistically too demanding. Some cultural values may also prove measurable, but may combine such complexity or inertia that they are inappropriate to monitor.
For some receptors, accurately measuring the baseline condition may be difficult, but monitoring changes may be feasible. For example, hydrological processes such as recharge of aquifers may be difficult to measure in absolute terms, but changes could be implicitly understood by monitoring aquifer levels (using observation bores), aquifer discharge to mound springs, or dry-season baseflow in streams.
It may prove impossible, impractical or unfeasible to measure or monitor receptors of certain asset classes. Such receptors may represent processes such as movement of water between subterranean aquifers, and some ecological phenomena that depend on climate and are thus stochastic.
5.1.2 Locations of potential exposure for species with a water-dependent phase in their life history
For those species that have a clearly understood water-dependent phase in their life history, there exists a range of possibilities for how and when they may potentially be exposed to changes in water quality or quantity due to CSG and coal mining development.
Some completely aquatic species will have life histories completely constrained to a single site, whether it is a spring, a wetland, a reach or some other discrete water body. Some terrestrial species that have limited ability to disperse but possess key dependencies on aquatic systems for food may also be explicitly linked to a single site.
For other aquatic species that move between water bodies within a catchment, only some parts of that catchment may be exposed to impacts from mining activities, but those impacts may be more widely felt indirectly or cumulatively through effects on, for example, breeding success or food webs. Most terrestrial species with direct reliance on aquatic systems for food will also fall into this category.
For species that spend some time in fresh water and some in marine systems, only the freshwater phase may be affected. For example, for catadromous fish like eels and barramundi, recruitment of fingerlings to maturity may be affected, even though breeding takes place at sea. Some terrestrial species may also be affected in such a way, particularly birds that range between marine and freshwater habitats. For species with only a transitory aquatic phase, such as many amphibians, the potential exposure may be limited both spatially and temporally. This is also true of species that are terrestrial yet have key dependencies on aquatic systems for parts of their life histories, for example, migratory birds that over-winter in wetland systems.
5.2 Impact analysis
The impact analysis (Component 3) requires an integrated approach to determine the direct, indirect and cumulative impacts of CSG and coal mining development. The impact analysis is not separate to the model-data analysis or risk analysis components but is integrally linked through the identified water-dependent assets and receptors.
The impact analysis is influenced by a number of factors that affect the method adopted. The methods should be practical and suitable for adoption in the bioregion under study given data, time and financial resources available. The nature of the impacts, the availability and quality of data, and the available capability and skills to undertake an analysis all influence the methods adopted. Decisions made should be documented in the workflow.
The concept of 'materiality' applies to the analysis of impacts on each receptor. It defines the magnitude and priority or scale of impacts on receptors. Those impacts regarded as immaterial are omitted from further analysis. In this way, efficient effort is directed towards the most important (i.e. material) receptors. Examples of material impacts on receptors might be:
- a loss of seasonal river flow volumes in n years out of 10 that eliminates breeding conditions for a particular arthropod (receptor: arthropod population in River X at location [x, y])
- declining pressure head on agricultural wells leading to x m reduction in pressure head and pumping flow rates that are less than 50% of current values (receptor: agricultural wells at location [x, y])
- a change in a water quality parameter that renders a habitat no longer suitable for a particular receptor (receptor: A particular species at location [x, y])
- an accumulation of salt concentration in agricultural soils from use of associated water leading to a significant (greater than X%) reduction in cereal crop production over 10 years (receptor: cropping land at location [x, y]).
The impact analysis relies on methods adopted and implemented by governments to assess the materiality of impacts on receptors. These methods include:
- expert opinion through consultation, workshops and panels
- consultation with stakeholders, including questionnaires
- checklists and matrices of impacts and causes
- spatial analysis using geographic information systems.
Natural resource management agencies have been requested to provide assets for input to BAs through the Office of Water for the priority bioregions. Once the water-dependent asset registers are provided by the natural resource management agencies, the following methods are used within the BA methodology for an impact analysis:
- network and systems analysis and conceptual models
- analysis of thresholds, carrying capacity and ecotoxicology
- numerical modelling of direct impacts
- numerical modelling of causal pathways to determine indirect and cumulative impacts
- conceptual modelling of causal pathways to determine indirect and cumulative impacts.
As part of Component 1 (contextual information) for a bioregion, baseline data are required for all receptors that are studied in the impact analysis. The acquisition of baseline data is ongoing and iterative, and is an integral part of the monitoring program extending beyond the current BAs. A correctly formulated monitoring program forms the basis for updating BAs. Data collection during monitoring should be cognisant of the development of the coal resource, the level of reduction in uncertainty, climate variation effects and impacts from other sectors.
5.2.1 Direct impacts
Direct impacts are those associated with CSG and coal mining developments that impact on natural resources without intervening agents or pathways. The direct impacts of CSG or coal mining development are the changes to the physical or chemical characteristics of groundwater and surface water resources that occur as a one-to-one result of dewatering of coal seams due to CSG or coal mining development. Examples of direct impacts are:
- the loss of pressure head in an aquifer as a result of the dewatering of a coal seam
- changes in groundwater chemistry due to a change in hydraulic relationships
- changes in hydraulic properties, such as porosity of an aquifer due to pressure reduction.
The results of direct impacts are estimated directly from numerical modelling of surface water and groundwater responding to current and future development pathways, and are expressed in units associated with state variables and parameters of these models. Uncertainties in direct impacts are derived from formal treatment of uncertainties in the numerical groundwater or surface water model. Where there are insufficient data, information or models, semi-quantitative or qualitative methods for assessing direct impacts may be adopted. Decisions, datasets and choices must be logged in the workflow.
Underground coal mining and extraction of water can cause subsidence of the land surface which can modify surface drainage, affecting land use. Common mining subsidence detection programs include global positioning system (GPS) surveys, conventional precise levelling and theodolite surveys, EDM surveys, and remote electronic monitoring (Hebblewhite et al., 2000). These techniques measure ground subsidence on a point-by-point basis and are, therefore, relatively time consuming and costly. Hence, they are usually constrained to localised areas, and it is very difficult to detect any regional deformation induced by underground mining or CSG extraction using these techniques. In the context of the regional-scale BAs, methods for detecting subsidence are most useful if they provide rapid assessment of spatial variations in subsidence rather than spot measurements. The tool best suited for this work is InSAR (Interferometric Synthetic Aperture Radar) using satellite platforms (Bürgmann et al., 2000). InSAR routinely detects land surface changes in the order of millimetres at rates of millimetres per year. This capability is available in Australia. InSAR requires repeated acquisition of satellite data, thus the method will require nomination of areas of concern for repeat surveys with sufficient time between surveys to detect changes. Other data, including LiDAR DEMs (Light Detection and Ranging-derived digital elevation models), are equally powerful datasets for detecting subsidence if repeat surveys are available. LiDAR can be very costly to acquire relative to InSAR; in cases where the data already exists, LiDAR DEMs may be appropriate to detect subsidence.
For specific, high-value features that may be affected by subsidence (such as infrastructure or important natural features), ground movement can be measured by Geodetic global positioning system (GPS) units. These units can measure millimetre-scale movement in the ground surface and rates of millimetres per year, if they are in place for a sufficient time, with the general rule that the smaller the rate of change, the longer it takes to measure it reliably. Geodetic GPS will be the preferred method for assessing subsidence if the soils in a location contain swelling clays. These soils can swell and shrink (Chertkov, 2005) at a level that can exceed the annual movement caused by mining or fluid extraction. These short-term natural movements could potentially mask subsidence in InSAR data.
5.2.2 Indirect impacts
Indirect impacts are those impacts on receptors (within water-dependent assets) that are produced as a result of a pathway of cause and effect. This causal pathway may be simple or complex. Sometimes indirect impacts are referred to as second- or third-level impacts, or secondary impacts (Walker and Johnston, 1999).
Indirect impacts of CSG or coal mining development are the effects on receptors that arise in direct response to the direct effects of coal seam dewatering. Examples of indirect impacts are:
- the effect of dewatering on a gaining or losing stream via the direct impact of loss of pressure head in an aquifer
- the drying of agricultural wells or natural springs
- salinisation of freshwater aquifers due to depressurisation of coal seams.
The results of indirect impacts are analysed through impact pathways which link the direct and indirect impacts on individual receptors defined in the receptor register for each water-dependent asset listed in the water-dependent asset register. The impact pathway may be derived as an explicit set of model functions that quantitatively relate the influence of direct impacts on the indirect impacts of individual receptors. This is the ideal approach and allows for a quantitative estimate of the magnitude of the indirect impacts. In addition, uncertainties in the numerical model can be propagated analytically or numerically through the impact analysis model to provide a quantitative level of confidence in the indirect impact. Alternatively, semi-quantitative or qualitative estimates of indirect impacts are to be determined where there is insufficient process knowledge or data to provide quantitative estimates of the indirect impacts. Decision points and course of action must be documented in the workflow.
5.2.3 Cumulative impacts of mining
Cumulative impacts are the aggregate, successive and incremental impacts on receptors, distributed in time and space, that occur in addition to the direct and indirect impacts of CSG and coal mining development. Cumulative impacts may be additive or multiplicative ('synergistic'), and are either positive or negative. They result from incremental changes caused by other past, present or reasonably foreseeable actions together with the CSG or coal mining development project. The interactions between impacts – whether between the impacts of just one project or between the impacts of other projects in the CSG and coal mining sectors – contribute to cumulative impacts (Walker and Johnston, 1999; Franks et al., 2010).
In the present context, cumulative impact analysis aims to identify the aggregate effects of CSG or coal mining development (in addition to the direct and indirect impacts identified in Section 5.2.1 and Section 5.2.2) on receptors within water-dependent assets, and to understand the response by regional groundwater and surface water resources to these impacts. This understanding then can assist with decisions that ensure the sustainable use of water resources. Cumulative impacts may result from the compounding effect of CSG and coal mining development from a single mine through time; multiple mines operating within a landscape; or legacy effects of past mining activities impacting on the present. They may also be generated where the distribution of CSG wells in a landscape affects – in an aggregate way – receptors that depend on groundwater and surface water.
Cumulative impacts aggregate in three ways (Franks et al., 2010):
- The interaction between spatially distributed impacts of different mine sites, or from multiple gas wells, may give rise to a cumulative impact greater than the sum of individual impacts from any single mine or well on its own.
- The accumulation of impacts through time may lead to synergistic effects that result in cumulative impacts emerging over time.
- Linkages between impacts may result in the triggering of further associated impacts that otherwise would not have occurred if such a threshold had not been exceeded.
The BA methodology requires an analysis of the cumulative impacts of CSG and coal mining developments. The cumulative impact analysis may be quantitative, semi-quantitative or qualitative depending on the level of confidence in data, information and models available and should build on and extend information obtained as part of the direct and indirect impact analysis (Section 5.2.1 and Section 5.2.2). Decisions regarding the type of analysis should be documented in the workflow.
The quantification of cumulative impacts can be difficult due to the complex nature of interactions among various components of a system that includes mining, agriculture, cities and the environment. As a result, the integrated approach outlined here in the BA methodology is necessary: first quantitatively assess the direct and indirect impacts, and then estimate the cumulative impacts that exist over and above the direct and indirect impacts. Cumulative impacts are receiving increasing attention by regulatory authorities and approval processes. As a result there is a pressing need to develop applied methodologies so as to streamline their analysis. It will become increasingly necessary for CSG and coal mining development projects to quantify and address the cumulative impacts in all regions of mining and petroleum sector activity whether previous activity by these sectors has been dense, sparse or absent.
The EPBC Act does not, in its present form, contain a specific requirement to assess cumulative impacts of development. However, Federal Court rulings have interpreted the EPBC Act to require an assessment of cumulative impacts of development proposals (Franks et al., 2010). Queensland and New South Wales state government Acts regarding environmental protection, infrastructure development and environmental impact statements require consideration of cumulative impacts. This assessment involves short- and long-term effects of development on the environment, visual amenity, air quality and water resources.
5.2.4 Cumulative impacts of other sectors
In addition to cumulative impacts within the mining sector, it is possible for cumulative impacts to be generated between sectors. For example, the operation of mining and irrigated agriculture within the same bioregion may generate cumulative impacts on groundwater and surface water resources that are in addition to the separate impacts of either agriculture or mining separately.
The BA methodology requires an estimate of the cumulative impacts of cross-sector interactions on water availability and use, and the associated probability. This estimate may be quantitative, semi-quantitative or qualitative depending on the level of understanding of the impacts and the complexity of interactions among multiple stakeholders. The regional scale of these assessments provides a basis for removing duplication of assessments at the level of the CSG and coal mining development projects, reducing the regulatory burden and providing greater certainty for development proposals. In order to understand the potential for cross-sector cumulative impacts, it is integral to refer to the contextual information, water balance assessment and the uncertainties in information. The risk analysis (Section 5.3) is to consider both the likelihood and consequence of these impacts.
5.3 Risk analysis
5.3.1 Background
Risk assessment and management are central components to any strategic management that seeks to identify and minimise threats while maximising opportunities. The IESC endorses the use of risk-based assessments as the best approach to providing scientific advice on proposed CSG and coal mining developments and their impacts on water-dependent assets. The aim of BAs will be to provide sufficient scientific advice to analyse the level of risk associated with impacts on water-dependent assets, particularly those of high value such as listed threatened species, state-listed wetlands and other important ecological and cultural water features. The BA methodology aims to ensure that the risk analysis work undertaken in a BA is compatible with further focused risk management projects within a bioregion. There are many approaches to assessing risks across the industrial, mining, environmental, agricultural, health, and safety sectors and thousands of published papers on methods and tools. To ensure consistency between risk-related work undertaken among different bioregions, the BA methodology will use AS/NZS ISO 31000:2009 Risk management – principles and guidelines. This standard builds on the earlier AS/NZS 4360:2004 standard. The ISO 31000:2009 standard uses a structured approach to identifying, defining, evaluating and treating risks. Monitoring, evaluation and stakeholder interactions are key components of best-practice risk management. The risk consequence table generated in the BAs (see Table 2) is a key point of stakeholder interaction with natural resource management agencies, state government agencies and community groups. The process to implement the ISO 31000:2009 standard is shown in Figure 4.
Figure 4. The risk management process, adapted from AS/NZS ISO 31000:2009 Risk management – principles and guidelines. The blue rectangle indicates the part of the process which will be implemented as part of the bioregional assessments
In the context of BAs and their purpose (namely to assist the IESC in providing advice on the likelihood of impacts of CSG and coal mining development on water-dependent assets), full application of the ISO 31000:2009 standard is outside scope, terms of reference and budget. However, in the context of providing risk-based scientific advice, it is important that any risk-related work undertaken within a BA is available to – and consistent with – other studies either underway or to be undertaken in the future. This consistency is provided by adoption of the ISO 31000:2009 standard.
The risk analysis undertaken within a BA is concerned only with water-related impacts on receptors contained within identified water-dependent assets. There is no consideration within a BA of the risks of CSG and coal mining development on vegetation, biodiversity, fauna or soils that have no water dependency and are not associated with assets.
This section of the BA methodology describes those components of the ISO 31000:2009 standard that are to be completed within a BA and those components that link to – but are outside of – the BA.
The purpose of assessing risk varies depending on use and on the individuals or organisations undertaking them. The purposes of applying risk assessment processes include Barry (2011):
- greater exploration and understanding of risks by a research team
- to meet an organisation's outcome requirement
- to ensure that a 'minimum level' of understanding of relative risks is achieved.
For the BA methodology, the purpose of the risk analysis is to:
- explore the issues of impact, likelihood and risk within a scientific and logical framework
- provide insights into where high-value water assets (for example water dependent listed threatened species and state-listed important water features) may face high risks
- identify where risks may occur that have previously not been identified or have been underestimated (i.e. surprises).
New knowledge on risks of CSG and coal mining development must be reported in the BA products.
5.3.2 AS/NZS ISO 31000:2009 Risk management – principles and guidelines
Risk is defined in the ISO 31000:2009 standard as 'the effect of uncertainty on objectives', with effect being 'a deviation from the expected' and uncertainty 'the state, even partial, of deficiency of information related to, understanding or knowledge of an event, its consequence, or likelihood'. This definition makes an important distinction between the 'event' and the 'effect' of that event. This distinction leads to a fundamental change in how risk is characterised in the new standard; that is, by the consequence of an event and the likelihood of that consequence rather than by the probability of the event itself (Purdy, 2009). Thus, the consequence of a risk occurring may be negative (hazard), positive (opportunity), or may, in fact, result in increased uncertainty (AIRMIC, Alarm and IRM, 2010). The ISO 31000:2009 standard also places strong emphasis on the setting of objectives within organisations that match community and stakeholder values, thereby necessitating considerable stakeholder interaction. In general, a risk assessment cannot therefore be conducted by isolated 'experts' acting independently of communities, industry and other stakeholders. The process involves stakeholder feedback and consultation at all stages through the risk assessment (Figure 4).
5.3.3 Risk analysis in the bioregional assessments
Risk analysis (Component 4) in the BAs is a key component that synthesises information from the impact analysis and model-data analysis components. It is important that:
- outputs from model-data analyses inform the direct, indirect and cumulative impacts which are used to define consequences required by the risk analysis
- outputs from propagation of uncertainties inform likelihoods required by the risk analysis.
The emphasis on rigorous assessment and propagation of uncertainty in models is key to their ability to provide robust scientific advice on risks. This represents a significant challenge and in many cases will require the use of qualitative or semi-quantitative approaches due to large uncertainties. Subsequent update and review of the BAs will lead to improved risk analyses in the future. However, where it is feasible, quantitative methods of risk analysis are preferred in a BA.
The BAs focus effort on the risk identification and risk analysis components of the ISO 31000:2009 standard (Figure 4):
- risk identification: Identification of risks within a bioregion begins with understanding the exposure of receptors to impacts from CSG and coal mining development and how this exposure may affect values of water-dependent assets. This includes taking into account existing mines and activities and risk mitigation and control measures already in place. A key component is the mapping of uncertainties associated with impacts on receptors to likelihoods of risks. This requires establishing clear and logical coupling between the impact analysis and risk analysis. It also requires a clear definition of what risk events exist within a bioregion informed by natural resource management agencies and their stakeholders. From this process, a risk register must be generated to inform the risk analysis.
- risk analysis: The risk analysis combines likelihood of event occurrence, uncertainties associated with impacts, and information from the risk register to generate (i) a consequence table (Table 3) describing the nature of impacts, and (ii) a risk rating matrix (Table 2) describing the severity of impacts. The consequence table will need to take into account receptor significance (for example, agricultural significance for an anthropogenic receptor or conservation ranking for an ecological receptor) in order to develop a robust measure of severity of impacts. The consequence table may contain social, cultural, historical, ceremonial or ecological consequences. Severity of impact will depend on who is affected by a risk. The risk rating matrix may be qualitative, semi-quantitative or quantitative depending on the degree of confidence in specifying events and their likelihood.
A key component to the analysis is the use of formal logic procedures to ensure that the assignment of likelihoods and consequences is transparent and logically consistent. In particular, developing correct conditional statements for the likelihood of events occurring (Barry, 2011) is critical to accurate risk analysis irrespective of whether they are qualitative or quantitative. Transparency means that qualitative descriptors are defined unambiguously and in a way that can be assessed and tested against other information sources either now or in the future. This will ensure that scientific advice is based on common assumptions and understandings and that the risk rating matrix is constructed in a rigorous way. Risk matrices can be unreliable and error prone leading to poor measures of risk exposure (Cox, 2008) unless careful attention is paid to: (i) the logical constructs and conditional probabilities of likelihoods used as input to the risk matrix; and (ii) clear definitions and care are used to determine severity of consequence. These are components of the risk analysis that require special attention in the BAs in each bioregion. Examples of these approaches are given in Barry (2011) and DRET (2008). The risk analyses must take into account the expected variation in surface water, groundwater and ecology of systems subject to impacts from CSG and coal mining development. This includes the impacts (both positive and negative) due to:
- depressurisation and dewatering of coal seams
- the regulated and unregulated discharge of worked water
- the release into the environment of associated CSG water or permeates and brines from treated associated water
- the recovery or recharge of coal seams (and associated connected aquifers) once dewatering has ceased.
It also refers to long-term impacts on anthropogenic and ecological receptors on the surface. Where surface water, groundwater and/or ecological systems move to a different state following dewatering and recovery, these states need to be characterised, likelihoods and consequences (risks) assessed, and uncertainties reported.
There are multiple quantitative approaches to dealing with uncertainty quantification, uncertainty propagation and risk analysis that can be used to assist with generating accurate quantitative estimates of likelihood of impacts on receptors. These include (Tartakovsky, 2013):
- probabilistic risk assessment methods and fault tree analyses for exposure pathways which are flexible and combine well with logic statements to generate conditional probabilities of events
- approaches based on random domain decomposition and transition probability for delineating boundaries from point observations
- Bayesian methods for calculating model structural uncertainties
- stochastic optimisation methods for quantifying propagation of uncertainties in parameters in models
- decision theory and 'optimisation-under-uncertainty' methods to assist with risk management and mitigation choices.
The particular methods to be applied in any bioregion will vary between bioregions based on characteristics of the geology, lithology, geomorphology, hydrology, ecology and coal development pathways.
Table 2. An example of a qualitative risk rating matrix (DRET, 2008) where consequence ratings range from 'insignificant' (1) to 'catastrophic' (5) and likelihoods range from 'rare' (E) to 'almost certain' (A). This is the minimal and simplest form of a risk rating matrix and is useful where consequences and likelihoods can be defined in qualitative terms
Likelihood level |
Consequence level |
||||
---|---|---|---|---|---|
1 - Insignificant |
2 - Minor |
3 - Moderate |
4 - Major |
5 - Catastrophic |
|
A - Almost certain |
A1 High |
A2 High |
A3 Extreme |
A4 Extreme |
A5 Extreme |
B - Likely |
B1 Moderate |
B2 High |
B3 High |
B4 Extreme |
B5 Extreme |
C - Possible |
C1 Low |
C2 Moderate |
C3 High |
C4 Extreme |
C5 Extreme |
D - Unlikely |
D1 Low |
D2 Low |
D3 Moderate |
D4 High |
D5 Extreme |
E - Rare |
E1 Low |
E2 Low |
E3 Moderate |
E4 High |
E5 High |
Table 3. An example of a consequence table (DRET, 2008) used to determine the consequences of impacts given certain specific events occurring. A consequence table must be developed for each bioregional assessment based on discussions with state natural resource management agencies and stakeholder local knowledge for consideration in the risk analysis component of an assessment
Impact category |
Consequence level |
||||
---|---|---|---|---|---|
Negligible 0.1 |
Minor 1 |
Moderate 10 |
Major 100 |
Extreme 1000 |
|
Property - Replacement cost |
$0-0.1M |
$0.1-1M |
$1-10M |
$10-100M |
$100-1000M |
Environment - Ecosystem function |
No detectable change |
Minor change |
Major change |
Irreversible change |
|
Environment - Habitat |
1% area |
1-5% area |
5-30% area |
30-90% area |
> 90% area |
Environment - Species |
Small population change |
Recovery, 1 year |
Recovery, 2 years |
Recovery, 10 years |
No recovery |
Health |
Minor injury |
Major injury |
Fatality |
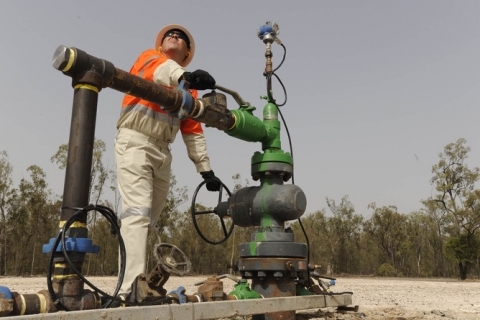